Cryo-EM structures of the archaeal PAN-proteasome reveal an around-the-ring ATPase cycle
- PMID: 30559193
- PMCID: PMC6329974
- DOI: 10.1073/pnas.1817752116
Cryo-EM structures of the archaeal PAN-proteasome reveal an around-the-ring ATPase cycle
Abstract
Proteasomes occur in all three domains of life, and are the principal molecular machines for the regulated degradation of intracellular proteins. They play key roles in the maintenance of protein homeostasis, and control vital cellular processes. While the eukaryotic 26S proteasome is extensively characterized, its putative evolutionary precursor, the archaeal proteasome, remains poorly understood. The primordial archaeal proteasome consists of a 20S proteolytic core particle (CP), and an AAA-ATPase module. This minimal complex degrades protein unassisted by non-ATPase subunits that are present in a 26S proteasome regulatory particle (RP). Using cryo-EM single-particle analysis, we determined structures of the archaeal CP in complex with the AAA-ATPase PAN (proteasome-activating nucleotidase). Five conformational states were identified, elucidating the functional cycle of PAN, and its interaction with the CP. Coexisting nucleotide states, and correlated intersubunit signaling features, coordinate rotation of the PAN-ATPase staircase, and allosterically regulate N-domain motions and CP gate opening. These findings reveal the structural basis for a sequential around-the-ring ATPase cycle, which is likely conserved in AAA-ATPases.
Keywords: ATPase cycle; PAN; archaea; cryo-EM; proteasome.
Copyright © 2019 the Author(s). Published by PNAS.
Conflict of interest statement
The authors declare no conflict of interest.
Figures
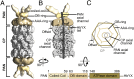
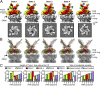
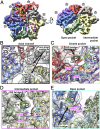
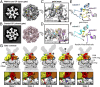
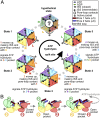
Comment in
-
Stepping up protein degradation.Proc Natl Acad Sci U S A. 2019 Jan 8;116(2):350-352. doi: 10.1073/pnas.1819949116. Epub 2018 Dec 19. Proc Natl Acad Sci U S A. 2019. PMID: 30567974 Free PMC article. No abstract available.
Similar articles
-
Structural insights into the functional cycle of the ATPase module of the 26S proteasome.Proc Natl Acad Sci U S A. 2017 Feb 7;114(6):1305-1310. doi: 10.1073/pnas.1621129114. Epub 2017 Jan 23. Proc Natl Acad Sci U S A. 2017. PMID: 28115689 Free PMC article.
-
Mechanism of gate opening in the 20S proteasome by the proteasomal ATPases.Mol Cell. 2008 May 9;30(3):360-8. doi: 10.1016/j.molcel.2008.03.004. Mol Cell. 2008. PMID: 18471981 Free PMC article.
-
An atomic model AAA-ATPase/20S core particle sub-complex of the 26S proteasome.Biochem Biophys Res Commun. 2009 Oct 16;388(2):228-33. doi: 10.1016/j.bbrc.2009.07.145. Epub 2009 Aug 3. Biochem Biophys Res Commun. 2009. PMID: 19653995 Free PMC article.
-
Proteasomes and their associated ATPases: a destructive combination.J Struct Biol. 2006 Oct;156(1):72-83. doi: 10.1016/j.jsb.2006.04.012. Epub 2006 May 8. J Struct Biol. 2006. PMID: 16919475 Review.
-
Proteasomal AAA-ATPases: structure and function.Biochim Biophys Acta. 2012 Jan;1823(1):67-82. doi: 10.1016/j.bbamcr.2011.07.009. Epub 2011 Jul 23. Biochim Biophys Acta. 2012. PMID: 21820014 Review.
Cited by
-
DiffModeler: large macromolecular structure modeling for cryo-EM maps using a diffusion model.Nat Methods. 2024 Dec;21(12):2307-2317. doi: 10.1038/s41592-024-02479-0. Epub 2024 Oct 21. Nat Methods. 2024. PMID: 39433880
-
The mycobacterial proteasomal ATPase Mpa forms a gapped ring to engage the 20S proteasome.J Biol Chem. 2021 Jan-Jun;296:100713. doi: 10.1016/j.jbc.2021.100713. Epub 2021 Apr 27. J Biol Chem. 2021. PMID: 33930464 Free PMC article.
-
Stoichiometry of Nucleotide Binding to Proteasome AAA+ ATPase Hexamer Established by Native Mass Spectrometry.Mol Cell Proteomics. 2020 Dec;19(12):1997-2015. doi: 10.1074/mcp.RA120.002067. Epub 2020 Sep 3. Mol Cell Proteomics. 2020. PMID: 32883800 Free PMC article.
-
Stepping up protein degradation.Proc Natl Acad Sci U S A. 2019 Jan 8;116(2):350-352. doi: 10.1073/pnas.1819949116. Epub 2018 Dec 19. Proc Natl Acad Sci U S A. 2019. PMID: 30567974 Free PMC article. No abstract available.
-
An empirical energy landscape reveals mechanism of proteasome in polypeptide translocation.Elife. 2022 Jan 20;11:e71911. doi: 10.7554/eLife.71911. Elife. 2022. PMID: 35050852 Free PMC article.
References
-
- Voges D, Zwickl P, Baumeister W. The 26S proteasome: A molecular machine designed for controlled proteolysis. Annu Rev Biochem. 1999;68:1015–1068. - PubMed
-
- Zwickl P, Baumeister W, Steven A. Dis-assembly lines: The proteasome and related ATPase-assisted proteases. Curr Opin Struct Biol. 2000;10:242–250. - PubMed
Publication types
MeSH terms
Substances
Associated data
- Actions
- Actions
- Actions
- Actions
- Actions
- Actions
- Actions
- Actions
LinkOut - more resources
Full Text Sources
Other Literature Sources
Miscellaneous