The AREB1 Transcription Factor Influences Histone Acetylation to Regulate Drought Responses and Tolerance in Populus trichocarpa
- PMID: 30538157
- PMCID: PMC6482633
- DOI: 10.1105/tpc.18.00437
The AREB1 Transcription Factor Influences Histone Acetylation to Regulate Drought Responses and Tolerance in Populus trichocarpa
Abstract
Plants develop tolerance to drought by activating genes with altered levels of epigenetic modifications. Specific transcription factors are involved in this activation, but the molecular connections within the regulatory system are unclear. Here, we analyzed genome-wide acetylated lysine residue 9 of histone H3 (H3K9ac) enrichment and examined its association with transcriptomes in Populus trichocarpa under drought stress. We revealed that abscisic acid-Responsive Element (ABRE) motifs in promoters of the drought-responsive genes PtrNAC006, PtrNAC007, and PtrNAC120 are involved in H3K9ac enhancement and activation of these genes. Overexpressing these PtrNAC genes in P trichocarpa resulted in strong drought-tolerance phenotypes. We showed that the ABRE binding protein PtrAREB1-2 binds to ABRE motifs associated with these PtrNAC genes and recruits the histone acetyltransferase unit ADA2b-GCN5, forming AREB1-ADA2b-GCN5 ternary protein complexes. Moreover, this recruitment enables GCN5-mediated histone acetylation to enhance H3K9ac and enrich RNA polymerase II specifically at these PtrNAC genes for the development of drought tolerance. CRISPR editing or RNA interference-mediated downregulation of any of the ternary members results in highly drought-sensitive P trichocarpa Thus, the combinatorial function of the ternary proteins establishes a coordinated histone acetylation and transcription factor-mediated gene activation for drought response and tolerance in Populus species.
© 2019 American Society of Plant Biologists. All rights reserved.
Figures
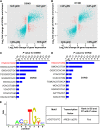
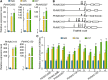
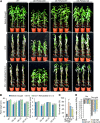
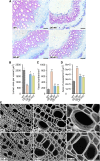
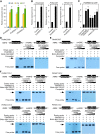
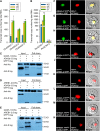
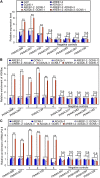
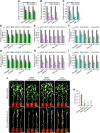
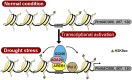
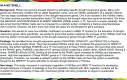
Comment in
-
The AREB1-ADA2b-GCN5 Complex Regulates Gene Expression during Drought Stress.Plant Cell. 2019 Mar;31(3):559-560. doi: 10.1105/tpc.18.00940. Epub 2018 Dec 20. Plant Cell. 2019. PMID: 30573471 Free PMC article. No abstract available.
Similar articles
-
Genome-wide identification and expression analysis of histone deacetylase and histone acetyltransferase genes in response to drought in poplars.BMC Genomics. 2024 Jul 2;25(1):657. doi: 10.1186/s12864-024-10570-1. BMC Genomics. 2024. PMID: 38956453 Free PMC article.
-
Improved drought stress tolerance in Arabidopsis by CRISPR/dCas9 fusion with a Histone AcetylTransferase.Sci Rep. 2019 May 30;9(1):8080. doi: 10.1038/s41598-019-44571-y. Sci Rep. 2019. PMID: 31147630 Free PMC article.
-
Alterations of lysine modifications on the histone H3 N-tail under drought stress conditions in Arabidopsis thaliana.Plant Cell Physiol. 2008 Oct;49(10):1580-8. doi: 10.1093/pcp/pcn133. Epub 2008 Sep 8. Plant Cell Physiol. 2008. PMID: 18779215
-
Updated Mechanisms of GCN5-The Monkey King of the Plant Kingdom in Plant Development and Resistance to Abiotic Stresses.Cells. 2021 Apr 22;10(5):979. doi: 10.3390/cells10050979. Cells. 2021. PMID: 33922251 Free PMC article. Review.
-
Histone Acetylation Changes in Plant Response to Drought Stress.Genes (Basel). 2021 Sep 13;12(9):1409. doi: 10.3390/genes12091409. Genes (Basel). 2021. PMID: 34573391 Free PMC article. Review.
Cited by
-
Ectopic Expression of PtrLBD39 Retarded Primary and Secondary Growth in Populus trichocarpa.Int J Mol Sci. 2024 Feb 12;25(4):2205. doi: 10.3390/ijms25042205. Int J Mol Sci. 2024. PMID: 38396881 Free PMC article.
-
Genome-Wide and Comprehensive Analysis of the Multiple Stress-Related CAF1 (CCR4-Associated Factor 1) Family and Its Expression in Poplar.Plants (Basel). 2021 May 14;10(5):981. doi: 10.3390/plants10050981. Plants (Basel). 2021. PMID: 34068989 Free PMC article.
-
Potassium indole-3-butyric acid affects rice's adaptability to salt stress by regulating carbon metabolism, transcription factor genes expression, and biosynthesis of secondary metabolites.Front Plant Sci. 2024 Sep 3;15:1416936. doi: 10.3389/fpls.2024.1416936. eCollection 2024. Front Plant Sci. 2024. PMID: 39290739 Free PMC article.
-
Histone Acetyltransferase SlGCN5 Regulates Shoot Meristem and Flower Development in Solanum lycopersicum.Front Plant Sci. 2022 Jan 21;12:805879. doi: 10.3389/fpls.2021.805879. eCollection 2021. Front Plant Sci. 2022. PMID: 35126431 Free PMC article.
-
Transcriptomic and Epigenomic Assessment Reveals Epigenetic Regulation of WRKY Genes in Response to Magnaporthe oryzae Infection in Rice.Curr Genomics. 2022 Jul 5;23(3):182-194. doi: 10.2174/1389202923666220510195910. Curr Genomics. 2022. PMID: 36777006 Free PMC article.
References
-
- Arango-Velez A., Zwiazek J.J., Thomas B.R., Tyree M.T. (2011). Stomatal factors and vulnerability of stem xylem to cavitation in poplars. Physiol. Plant. 143: 154–165. - PubMed
-
- Ascenzi R., Gantt J.S. (1999). Molecular genetic analysis of the drought-inducible linker histone variant in Arabidopsis thaliana. Plant Mol. Biol. 41: 159–169. - PubMed
-
- Aubert Y., Vile D., Pervent M., Aldon D., Ranty B., Simonneau T., Vavasseur A., Galaud J.P. (2010). RD20, a stress-inducible caleosin, participates in stomatal control, transpiration and drought tolerance in Arabidopsis thaliana. Plant Cell Physiol. 51: 1975–1987. - PubMed
-
- Balasubramanian R., Pray-Grant M.G., Selleck W., Grant P.A., Tan S. (2002). Role of the Ada2 and Ada3 transcriptional coactivators in histone acetylation. J. Biol. Chem. 277: 7989–7995. - PubMed
-
- Barber V.A., Juday G.P., Finney B.P. (2000). Reduced growth of Alaskan white spruce in the twentieth century from temperature-induced drought stress. Nature 405: 668–673. - PubMed
Publication types
MeSH terms
Substances
LinkOut - more resources
Full Text Sources
Molecular Biology Databases