Anthelmintic drug actions in resistant and susceptible C. elegans revealed by electrophysiological recordings in a multichannel microfluidic device
- PMID: 30503202
- PMCID: PMC6287544
- DOI: 10.1016/j.ijpddr.2018.10.003
Anthelmintic drug actions in resistant and susceptible C. elegans revealed by electrophysiological recordings in a multichannel microfluidic device
Abstract
Many anthelmintic drugs used to treat parasitic nematode infections target proteins that regulate electrical activity of neurons and muscles: ion channels (ICs) and neurotransmitter receptors (NTRs). Perturbation of IC/NTR function disrupts worm behavior and can lead to paralysis, starvation, immune attack and expulsion. Limitations of current anthelmintics include a limited spectrum of activity across species and the threat of drug resistance, highlighting the need for new drugs for human and veterinary medicine. Although ICs/NTRs are valuable anthelmintic targets, electrophysiological recordings are not commonly included in drug development pipelines. We designed a medium-throughput platform for recording electropharyngeograms (EPGs)-the electrical signals emitted by muscles and neurons of the pharynx during pharyngeal pumping (feeding)-in Caenorhabditis elegans and parasitic nematodes. The current study in C. elegans expands previous work in several ways. Detecting anthelmintic bioactivity in drugs, compounds or natural products requires robust, sustained pharyngeal pumping under baseline conditions. We generated concentration-response curves for stimulating pumping by perfusing 8-channel microfluidic devices (chips) with the neuromodulator serotonin, or with E. coli bacteria (C. elegans' food in the laboratory). Worm orientation in the chip (head-first vs. tail-first) affected the response to E. coli but not to serotonin. Using a panel of anthelmintics-ivermectin, levamisole and piperazine-targeting different ICs/NTRs, we determined the effects of concentration and treatment duration on EPG activity, and successfully distinguished control (N2) and drug-resistant worms (avr-14; avr-15; glc-1, unc-38 and unc-49). EPG recordings detected anthelmintic activity of drugs that target ICs/NTRs located in the pharynx as well as at extra-pharyngeal sites. A bus-8 mutant with enhanced permeability was more sensitive than controls to drug treatment. These results provide a useful framework for investigators who would like to more easily incorporate electrophysiology as a routine component of their anthelmintic research workflow.
Keywords: Anthelmintic drug; C. elegans; Drug screening; Electrophysiology; Microfluidics; Pharyngeal pumping.
Copyright © 2018 The Authors. Published by Elsevier Ltd.. All rights reserved.
Figures
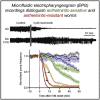
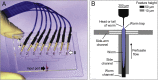
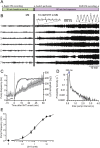
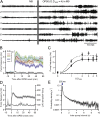
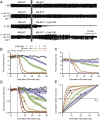
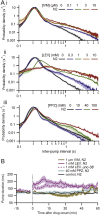
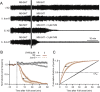
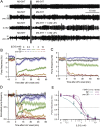
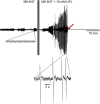
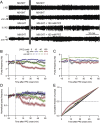
Similar articles
-
Comparison of electrophysiological and motility assays to study anthelmintic effects in Caenorhabditis elegans.Int J Parasitol Drugs Drug Resist. 2021 Aug;16:174-187. doi: 10.1016/j.ijpddr.2021.05.005. Epub 2021 Jul 2. Int J Parasitol Drugs Drug Resist. 2021. PMID: 34252686 Free PMC article.
-
Microfluidic platform for electrophysiological recordings from host-stage hookworm and Ascaris suum larvae: A new tool for anthelmintic research.Int J Parasitol Drugs Drug Resist. 2016 Dec;6(3):314-328. doi: 10.1016/j.ijpddr.2016.08.001. Epub 2016 Sep 15. Int J Parasitol Drugs Drug Resist. 2016. PMID: 27751868 Free PMC article.
-
Deciphering the molecular determinants of cholinergic anthelmintic sensitivity in nematodes: When novel functional validation approaches highlight major differences between the model Caenorhabditis elegans and parasitic species.PLoS Pathog. 2018 May 2;14(5):e1006996. doi: 10.1371/journal.ppat.1006996. eCollection 2018 May. PLoS Pathog. 2018. PMID: 29719008 Free PMC article.
-
Pharmacology of anthelmintic resistance.Parasitology. 1996;113 Suppl:S201-16. doi: 10.1017/s0031182000077982. Parasitology. 1996. PMID: 9051936 Review.
-
Cyclooctadepsipeptides--an anthelmintically active class of compounds exhibiting a novel mode of action.Int J Antimicrob Agents. 2003 Sep;22(3):318-31. doi: 10.1016/s0924-8579(03)00219-x. Int J Antimicrob Agents. 2003. PMID: 13678839 Review.
Cited by
-
Comparison of electrophysiological and motility assays to study anthelmintic effects in Caenorhabditis elegans.Int J Parasitol Drugs Drug Resist. 2021 Aug;16:174-187. doi: 10.1016/j.ijpddr.2021.05.005. Epub 2021 Jul 2. Int J Parasitol Drugs Drug Resist. 2021. PMID: 34252686 Free PMC article.
-
-Using the C. elegans lem-2 Gene to Reconstruct the Human LEMD2 Mutation Associated with Hutterite-type Cataract/Cardiomyopathy.MicroPubl Biol. 2020 Jun 29;2020:10.17912/micropub.biology.000273. doi: 10.17912/micropub.biology.000273. MicroPubl Biol. 2020. PMID: 32666044 Free PMC article. No abstract available.
-
Pharyngeal Pumping and Tissue-Specific Transgenic P-Glycoprotein Expression Influence Macrocyclic Lactone Susceptibility in Caenorhabditis elegans.Pharmaceuticals (Basel). 2021 Feb 13;14(2):153. doi: 10.3390/ph14020153. Pharmaceuticals (Basel). 2021. PMID: 33668460 Free PMC article.
-
Pharmacological Profiling of a Brugia malayi Muscarinic Acetylcholine Receptor as a Putative Antiparasitic Target.Antimicrob Agents Chemother. 2023 Jan 24;67(1):e0118822. doi: 10.1128/aac.01188-22. Epub 2023 Jan 5. Antimicrob Agents Chemother. 2023. PMID: 36602350 Free PMC article.
-
Caenorhabditis elegans in anthelmintic research - Old model, new perspectives.Int J Parasitol Drugs Drug Resist. 2020 Dec;14:237-248. doi: 10.1016/j.ijpddr.2020.09.005. Epub 2020 Oct 2. Int J Parasitol Drugs Drug Resist. 2020. PMID: 33249235 Free PMC article. Review.
References
-
- Abongwa M., Buxton S.K., Courtot E., Charvet C.L., Neveu C., McCoy C.J., Verma S., Robertson A.P., Martin R.J. Pharmacological profile of Ascaris suum ACR‐16, a new homomeric nicotinic acetylcholine receptor widely distributed in Ascaris tissues. Br. J. Pharmacol. 2016;173:2463–2477. doi: 10.1111/bph.13524. - DOI - PMC - PubMed
-
- Avery L. Motor neuron M3 controls pharyngeal muscle relaxation timing in Caenorhabditis elegans. J. Exp. Biol. 1993;175:283–297. - PubMed
Publication types
MeSH terms
Substances
Grants and funding
LinkOut - more resources
Full Text Sources