Orchestration of protein acetylation as a toggle for cellular defense and virus replication
- PMID: 30470744
- PMCID: PMC6251895
- DOI: 10.1038/s41467-018-07179-w
Orchestration of protein acetylation as a toggle for cellular defense and virus replication
Abstract
Emerging evidence highlights protein acetylation, a prevalent lysine posttranslational modification, as a regulatory mechanism and promising therapeutic target in human viral infections. However, how infections dynamically alter global cellular acetylation or whether viral proteins are acetylated remains virtually unexplored. Here, we establish acetylation as a highly-regulated molecular toggle of protein function integral to the herpesvirus human cytomegalovirus (HCMV) replication. We offer temporal resolution of cellular and viral acetylations. By interrogating dynamic protein acetylation with both protein abundance and subcellular localization, we discover finely tuned spatial acetylations across infection time. We determine that lamin acetylation at the nuclear periphery protects against virus production by inhibiting capsid nuclear egress. Further studies within infectious viral particles identify numerous acetylations, including on the viral transcriptional activator pUL26, which we show represses virus production. Altogether, this study provides specific insights into functions of cellular and viral protein acetylations and a valuable resource of dynamic acetylation events.
Conflict of interest statement
The authors declare no Competing Interests.
Figures
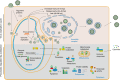
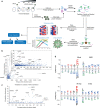
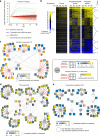
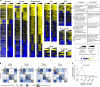
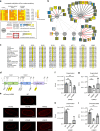
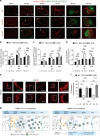
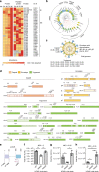
Similar articles
-
Identification of Host Factors Involved in Human Cytomegalovirus Replication, Assembly, and Egress Using a Two-Step Small Interfering RNA Screen.mBio. 2018 Jun 26;9(3):e00716-18. doi: 10.1128/mBio.00716-18. mBio. 2018. PMID: 29946045 Free PMC article.
-
Human cytomegalovirus riding the cell cycle.Med Microbiol Immunol. 2015 Jun;204(3):409-19. doi: 10.1007/s00430-015-0396-z. Epub 2015 Mar 17. Med Microbiol Immunol. 2015. PMID: 25776080 Review.
-
Novel mode of phosphorylation-triggered reorganization of the nuclear lamina during nuclear egress of human cytomegalovirus.J Biol Chem. 2010 Apr 30;285(18):13979-89. doi: 10.1074/jbc.M109.063628. Epub 2010 Mar 4. J Biol Chem. 2010. PMID: 20202933 Free PMC article.
-
Human Cytomegalovirus Nuclear Capsids Associate with the Core Nuclear Egress Complex and the Viral Protein Kinase pUL97.Viruses. 2018 Jan 13;10(1):35. doi: 10.3390/v10010035. Viruses. 2018. PMID: 29342872 Free PMC article.
-
Human Cytomegalovirus Strategies to Maintain and Promote mRNA Translation.Viruses. 2016 Apr 13;8(4):97. doi: 10.3390/v8040097. Viruses. 2016. PMID: 27089357 Free PMC article. Review.
Cited by
-
Virus-host protein interactions as footprints of human cytomegalovirus replication.Curr Opin Virol. 2022 Feb;52:135-147. doi: 10.1016/j.coviro.2021.11.016. Epub 2021 Dec 16. Curr Opin Virol. 2022. PMID: 34923282 Free PMC article. Review.
-
Identification of Lysine Acetylation Sites on MERS-CoV Replicase pp1ab.Mol Cell Proteomics. 2020 Aug;19(8):1303-1309. doi: 10.1074/mcp.RA119.001897. Epub 2020 May 18. Mol Cell Proteomics. 2020. PMID: 32424026 Free PMC article.
-
Target Discovery for Host-Directed Antiviral Therapies: Application of Proteomics Approaches.mSystems. 2021 Oct 26;6(5):e0038821. doi: 10.1128/mSystems.00388-21. Epub 2021 Sep 14. mSystems. 2021. PMID: 34519533 Free PMC article.
-
Targeting the Host Mitochondria as a Novel Human Cytomegalovirus Antiviral Strategy.Viruses. 2023 Apr 28;15(5):1083. doi: 10.3390/v15051083. Viruses. 2023. PMID: 37243170 Free PMC article. Review.
-
TRANSPIRE: A Computational Pipeline to Elucidate Intracellular Protein Movements from Spatial Proteomics Data Sets.J Am Soc Mass Spectrom. 2020 Jul 1;31(7):1422-1439. doi: 10.1021/jasms.0c00033. Epub 2020 May 29. J Am Soc Mass Spectrom. 2020. PMID: 32401031 Free PMC article.
References
Publication types
MeSH terms
Substances
Grants and funding
LinkOut - more resources
Full Text Sources
Other Literature Sources
Medical
Research Materials