Transcription Factors Activate Genes through the Phase-Separation Capacity of Their Activation Domains
- PMID: 30449618
- PMCID: PMC6295254
- DOI: 10.1016/j.cell.2018.10.042
Transcription Factors Activate Genes through the Phase-Separation Capacity of Their Activation Domains
Abstract
Gene expression is controlled by transcription factors (TFs) that consist of DNA-binding domains (DBDs) and activation domains (ADs). The DBDs have been well characterized, but little is known about the mechanisms by which ADs effect gene activation. Here, we report that diverse ADs form phase-separated condensates with the Mediator coactivator. For the OCT4 and GCN4 TFs, we show that the ability to form phase-separated droplets with Mediator in vitro and the ability to activate genes in vivo are dependent on the same amino acid residues. For the estrogen receptor (ER), a ligand-dependent activator, we show that estrogen enhances phase separation with Mediator, again linking phase separation with gene activation. These results suggest that diverse TFs can interact with Mediator through the phase-separating capacity of their ADs and that formation of condensates with Mediator is involved in gene activation.
Keywords: activation domain; gene activation; mediator; phase separation; transcription; transcription factor.
Copyright © 2018 Elsevier Inc. All rights reserved.
Figures
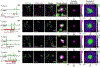
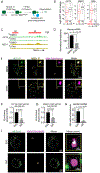
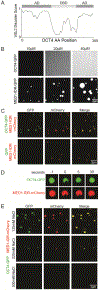
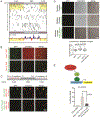
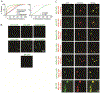
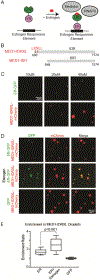
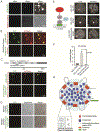
Comment in
-
Phase Separation, Protein Disorder, and Enhancer Function.Cell. 2018 Dec 13;175(7):1723-1725. doi: 10.1016/j.cell.2018.11.034. Cell. 2018. PMID: 30550782
Similar articles
-
Esrrb extinction triggers dismantling of naïve pluripotency and marks commitment to differentiation.EMBO J. 2018 Nov 2;37(21):e95476. doi: 10.15252/embj.201695476. Epub 2018 Oct 1. EMBO J. 2018. PMID: 30275266 Free PMC article.
-
CHIR99021 enhances Klf4 Expression through β-Catenin Signaling and miR-7a Regulation in J1 Mouse Embryonic Stem Cells.PLoS One. 2016 Mar 3;11(3):e0150936. doi: 10.1371/journal.pone.0150936. eCollection 2016. PLoS One. 2016. PMID: 26938105 Free PMC article.
-
Simple biochemical features underlie transcriptional activation domain diversity and dynamic, fuzzy binding to Mediator.Elife. 2021 Apr 27;10:e68068. doi: 10.7554/eLife.68068. Elife. 2021. PMID: 33904398 Free PMC article.
-
Esrrb activates Oct4 transcription and sustains self-renewal and pluripotency in embryonic stem cells.J Biol Chem. 2008 Dec 19;283(51):35825-33. doi: 10.1074/jbc.M803481200. Epub 2008 Oct 28. J Biol Chem. 2008. PMID: 18957414
-
Transcriptional activation of genes by 17 beta-estradiol through estrogen receptor-Sp1 interactions.Vitam Horm. 2001;62:231-52. doi: 10.1016/s0083-6729(01)62006-5. Vitam Horm. 2001. PMID: 11345900 Review.
Cited by
-
3D reconstruction of genomic regions from sparse interaction data.NAR Genom Bioinform. 2021 Mar 22;3(1):lqab017. doi: 10.1093/nargab/lqab017. eCollection 2021 Mar. NAR Genom Bioinform. 2021. PMID: 33778492 Free PMC article.
-
Longer DNA exhibits greater potential for cell-free gene expression.Sci Rep. 2021 Jun 3;11(1):11739. doi: 10.1038/s41598-021-91243-x. Sci Rep. 2021. PMID: 34083658 Free PMC article.
-
Phase separation of Arabidopsis EMB1579 controls transcription, mRNA splicing, and development.PLoS Biol. 2020 Jul 21;18(7):e3000782. doi: 10.1371/journal.pbio.3000782. eCollection 2020 Jul. PLoS Biol. 2020. PMID: 32692742 Free PMC article.
-
Effect of RNA on Morphology and Dynamics of Membraneless Organelles.J Phys Chem B. 2021 May 20;125(19):5035-5044. doi: 10.1021/acs.jpcb.1c02286. Epub 2021 May 10. J Phys Chem B. 2021. PMID: 33969989 Free PMC article.
-
Single-cell multiomics reveals the complexity of TGFβ signalling to chromatin in iPSC-derived kidney organoids.Commun Biol. 2022 Nov 27;5(1):1301. doi: 10.1038/s42003-022-04264-1. Commun Biol. 2022. PMID: 36435939 Free PMC article.
References
-
- Alberti S (2017). The wisdom of crowds: regulating cell function through condensed states of living matter. J. Cell Sci. 130, 2789–2796. - PubMed
-
- Arany Z, Newsome D, Oldread E, Livingston DM, and Eckner R (1995). A family of transcriptional adaptor proteins targeted by the E1A oncoprotein. Nature 374, 81–84. - PubMed
Publication types
MeSH terms
Substances
Grants and funding
LinkOut - more resources
Full Text Sources
Other Literature Sources
Molecular Biology Databases