Nε-lysine acetylation in the endoplasmic reticulum - a novel cellular mechanism that regulates proteostasis and autophagy
- PMID: 30446507
- PMCID: PMC6262770
- DOI: 10.1242/jcs.221747
Nε-lysine acetylation in the endoplasmic reticulum - a novel cellular mechanism that regulates proteostasis and autophagy
Abstract
Protein post-translational modifications (PTMs) take many shapes, have many effects and are necessary for cellular homeostasis. One of these PTMs, Nε-lysine acetylation, was thought to occur only in the mitochondria, cytosol and nucleus, but this paradigm was challenged in the past decade with the discovery of lysine acetylation in the lumen of the endoplasmic reticulum (ER). This process is governed by the ER acetylation machinery: the cytosol:ER-lumen acetyl-CoA transporter AT-1 (also known as SLC33A1), and the ER-resident lysine acetyltransferases ATase1 and ATase2 (also known as NAT8B and NAT8, respectively). This Review summarizes the more recent biochemical, cellular and mouse model studies that underscore the importance of the ER acetylation process in maintaining protein homeostasis and autophagy within the secretory pathway, and its impact on developmental and age-associated diseases.
Keywords: Autophagy; Endoplasmic reticulum; Lysine acetylation; Secretory pathway.
© 2018. Published by The Company of Biologists Ltd.
Conflict of interest statement
Competing interestsThe authors declare no competing or financial interests.
Figures
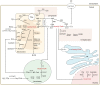
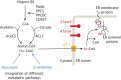
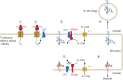
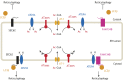
Similar articles
-
The endoplasmic reticulum acetyltransferases ATase1/NAT8B and ATase2/NAT8 are differentially regulated to adjust engagement of the secretory pathway.J Neurochem. 2020 Aug;154(4):404-423. doi: 10.1111/jnc.14958. Epub 2020 Jan 27. J Neurochem. 2020. PMID: 31945187 Free PMC article.
-
Nϵ-lysine acetylation in the lumen of the endoplasmic reticulum: A way to regulate autophagy and maintain protein homeostasis in the secretory pathway.Autophagy. 2016 Jun 2;12(6):1051-2. doi: 10.1080/15548627.2016.1164369. Epub 2016 Apr 28. Autophagy. 2016. PMID: 27124586 Free PMC article.
-
Improved proteostasis in the secretory pathway rescues Alzheimer's disease in the mouse.Brain. 2016 Mar;139(Pt 3):937-52. doi: 10.1093/brain/awv385. Epub 2016 Jan 19. Brain. 2016. PMID: 26787453 Free PMC article.
-
Lysine acetylation in the lumen of the ER: a novel and essential function under the control of the UPR.Biochim Biophys Acta. 2013 Mar;1833(3):686-97. doi: 10.1016/j.bbamcr.2012.12.004. Epub 2012 Dec 13. Biochim Biophys Acta. 2013. PMID: 23247107 Free PMC article. Review.
-
Intracellular Citrate/acetyl-CoA flux and endoplasmic reticulum acetylation: Connectivity is the answer.Mol Metab. 2023 Jan;67:101653. doi: 10.1016/j.molmet.2022.101653. Epub 2022 Dec 10. Mol Metab. 2023. PMID: 36513219 Free PMC article. Review.
Cited by
-
SLC13A5/sodium-citrate co-transporter overexpression causes disrupted white matter integrity and an autistic-like phenotype.Brain Commun. 2022 Jan 5;4(1):fcac002. doi: 10.1093/braincomms/fcac002. eCollection 2022 Feb. Brain Commun. 2022. PMID: 35146426 Free PMC article.
-
Lysine Acetyltransferase p300/CBP Plays an Important Role in Reproduction, Embryogenesis and Longevity of the Pea Aphid Acyrthosiphon pisum.Insects. 2020 Apr 26;11(5):265. doi: 10.3390/insects11050265. Insects. 2020. PMID: 32357443 Free PMC article.
-
ATG9A regulates proteostasis through reticulophagy receptors FAM134B and SEC62 and folding chaperones CALR and HSPB1.iScience. 2021 Mar 16;24(4):102315. doi: 10.1016/j.isci.2021.102315. eCollection 2021 Apr 23. iScience. 2021. PMID: 33870132 Free PMC article.
-
A Molecular Perspective on Sirtuin Activity.Int J Mol Sci. 2020 Nov 15;21(22):8609. doi: 10.3390/ijms21228609. Int J Mol Sci. 2020. PMID: 33203121 Free PMC article. Review.
-
Deficient Endoplasmic Reticulum Acetyl-CoA Import in Pancreatic Acinar Cells Leads to Chronic Pancreatitis.Cell Mol Gastroenterol Hepatol. 2021;11(3):725-738. doi: 10.1016/j.jcmgh.2020.10.008. Epub 2020 Oct 17. Cell Mol Gastroenterol Hepatol. 2021. PMID: 33080365 Free PMC article.
References
-
- Axe E. L., Walker S. A., Manifava M., Chandra P., Roderick H. L., Habermann A., Griffiths G. and Ktistakis N. T. (2008). Autophagosome formation from membrane compartments enriched in phosphatidylinositol 3-phosphate and dynamically connected to the endoplasmic reticulum. J. Cell Biol. 182, 685-701. 10.1083/jcb.200803137 - DOI - PMC - PubMed
Publication types
MeSH terms
Substances
Grants and funding
LinkOut - more resources
Full Text Sources
Research Materials
Miscellaneous