Functional Characterization of Two Class II Diterpene Synthases Indicates Additional Specialized Diterpenoid Pathways in Maize (Zea mays)
- PMID: 30405674
- PMCID: PMC6206430
- DOI: 10.3389/fpls.2018.01542
Functional Characterization of Two Class II Diterpene Synthases Indicates Additional Specialized Diterpenoid Pathways in Maize (Zea mays)
Abstract
As a major staple food, maize (Zea mays) is critical to food security. Shifting environmental pressures increasingly hamper crop defense capacities, causing expanded harvest loss. Specialized labdane-type diterpenoids are key components of maize chemical defense and ecological adaptation. Labdane diterpenoid biosynthesis most commonly requires the pairwise activity of class II and class I diterpene synthases (diTPSs) that convert the central precursor geranylgeranyl diphosphate into distinct diterpenoid scaffolds. Two maize class II diTPSs, ANTHER EAR 1 and 2 (ZmAN1/2), have been previously identified as catalytically redundant ent-copalyl diphosphate (CPP) synthases. ZmAN1 is essential for gibberellin phytohormone biosynthesis, whereas ZmAN2 is stress-inducible and governs the formation of defensive kauralexin and dolabralexin diterpenoids. Here, we report the biochemical characterization of the two remaining class II diTPSs present in the maize genome, COPALYL DIPHOSPHATE SYNTHASE 3 (ZmCPS3) and COPALYL DIPHOSPHATE SYNTHASE 4 (ZmCPS4). Functional analysis via microbial co-expression assays identified ZmCPS3 as a (+)-CPP synthase, with functionally conserved orthologs occurring in wheat (Triticum aestivum) and numerous dicot species. ZmCPS4 formed the unusual prenyl diphosphate, 8,13-CPP (labda-8,13-dien-15-yl diphosphate), as verified by mass spectrometry and nuclear magnetic resonance. As a minor product, ZmCPS4 also produced labda-13-en-8-ol diphosphate (LPP). Root gene expression profiles did not indicate an inducible role of ZmCPS3 in maize stress responses. By contrast, ZmCPS4 showed a pattern of inducible gene expression in roots exposed to oxidative stress, supporting a possible role in abiotic stress responses. Identification of the catalytic activities of ZmCPS3 and ZmCPS4 clarifies the first committed reactions controlling the diversity of defensive diterpenoids in maize, and suggests the existence of additional yet undiscovered diterpenoid pathways.
Keywords: Zea mays; diterpene synthase; diterpenoid biosynthesis; plant specialized metabolism; plant stress response.
Figures
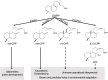
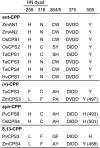
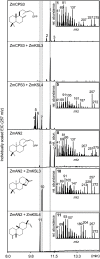
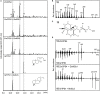
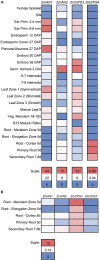
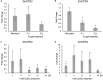
Similar articles
-
Functional Diversity of Diterpene Synthases in the Biofuel Crop Switchgrass.Plant Physiol. 2018 Sep;178(1):54-71. doi: 10.1104/pp.18.00590. Epub 2018 Jul 15. Plant Physiol. 2018. PMID: 30008447 Free PMC article.
-
Exploring diterpene metabolism in non-model species: transcriptome-enabled discovery and functional characterization of labda-7,13E-dienyl diphosphate synthase from Grindelia robusta.Plant J. 2015 Sep;83(5):783-93. doi: 10.1111/tpj.12925. Epub 2015 Jul 23. Plant J. 2015. PMID: 26119826
-
Discovery and characterization of diterpene synthases in Chamaecyparis formosensis Matsum. which participated in an unprecedented diterpenoid biosynthesis route in conifer.Plant Sci. 2021 Mar;304:110790. doi: 10.1016/j.plantsci.2020.110790. Epub 2020 Dec 9. Plant Sci. 2021. PMID: 33568294
-
Evolution of Labdane-Related Diterpene Synthases in Cereals.Plant Cell Physiol. 2020 Dec 23;61(11):1850-1859. doi: 10.1093/pcp/pcaa106. Plant Cell Physiol. 2020. PMID: 32810270 Review.
-
Specialized diterpenoid metabolism in monocot crops: Biosynthesis and chemical diversity.Phytochemistry. 2020 Apr;172:112289. doi: 10.1016/j.phytochem.2020.112289. Epub 2020 Feb 6. Phytochemistry. 2020. PMID: 32036187 Review.
Cited by
-
Stressing the importance of plant specialized metabolites: omics-based approaches for discovering specialized metabolism in plant stress responses.Front Plant Sci. 2023 Nov 8;14:1272363. doi: 10.3389/fpls.2023.1272363. eCollection 2023. Front Plant Sci. 2023. PMID: 38023861 Free PMC article. Review.
-
Specialized metabolites as mediators for plant-fungus crosstalk and their evolving roles.Curr Opin Plant Biol. 2021 Dec;64:102141. doi: 10.1016/j.pbi.2021.102141. Epub 2021 Nov 20. Curr Opin Plant Biol. 2021. PMID: 34814027 Free PMC article. Review.
-
Building a custom high-throughput platform at the Joint Genome Institute for DNA construct design and assembly-present and future challenges.Synth Biol (Oxf). 2020 Nov 5;5(1):ysaa023. doi: 10.1093/synbio/ysaa023. eCollection 2020. Synth Biol (Oxf). 2020. PMID: 34746437 Free PMC article.
-
Conserved bases for the initial cyclase in gibberellin biosynthesis: from bacteria to plants.Biochem J. 2019 Sep 24;476(18):2607-2621. doi: 10.1042/BCJ20190479. Biochem J. 2019. PMID: 31484677 Free PMC article.
-
A dolabralexin-deficient mutant provides insight into specialized diterpenoid metabolism in maize.Plant Physiol. 2023 May 31;192(2):1338-1358. doi: 10.1093/plphys/kiad150. Plant Physiol. 2023. PMID: 36896653 Free PMC article.
References
-
- Caniard A., Zerbe P., Legrand S., Cohade A., Valot N., Magnard J. L., et al. (2012). Discovery and functional characterization of two diterpene synthases for sclareol biosynthesis in Salvia sclarea (L.) and their relevance for perfume manufacture. BMC Plant Biol. 12:119. 10.1186/1471-2229-12-119 - DOI - PMC - PubMed
-
- Chaturvedi R., Venables B., Petros R. A., Nalam V., Li M., Wang X., et al. (2012). An abietane diterpenoid is a potent activator of systemic acquired resistance. Plant J. 71 161–172. - PubMed
LinkOut - more resources
Full Text Sources
Other Literature Sources
Research Materials
Miscellaneous