Dual cholinergic signals regulate daily migration of hematopoietic stem cells and leukocytes
- PMID: 30361261
- PMCID: PMC6449569
- DOI: 10.1182/blood-2018-08-867648
Dual cholinergic signals regulate daily migration of hematopoietic stem cells and leukocytes
Erratum in
-
García-García A, Korn C, García-Fernández M, et al. Dual cholinergic signals regulate daily migration of hematopoietic stem cells and leukocytes. Blood. 2019;133(3):224-236.Blood. 2020 Dec 17;136(25):2965. doi: 10.1182/blood.2020009650. Blood. 2020. PMID: 33331935 Free PMC article. No abstract available.
Abstract
Hematopoietic stem and progenitor cells (HSPCs) and leukocytes circulate between the bone marrow (BM) and peripheral blood following circadian oscillations. Autonomic sympathetic noradrenergic signals have been shown to regulate HSPC and leukocyte trafficking, but the role of the cholinergic branch has remained unexplored. We have investigated the role of the cholinergic nervous system in the regulation of day/night traffic of HSPCs and leukocytes in mice. We show here that the autonomic cholinergic nervous system (including parasympathetic and sympathetic) dually regulates daily migration of HSPCs and leukocytes. At night, central parasympathetic cholinergic signals dampen sympathetic noradrenergic tone and decrease BM egress of HSPCs and leukocytes. However, during the daytime, derepressed sympathetic noradrenergic activity causes predominant BM egress of HSPCs and leukocytes via β3-adrenergic receptor. This egress is locally supported by light-triggered sympathetic cholinergic activity, which inhibits BM vascular cell adhesion and homing. In summary, central (parasympathetic) and local (sympathetic) cholinergic signals regulate day/night oscillations of circulating HSPCs and leukocytes. This study shows how both branches of the autonomic nervous system cooperate to orchestrate daily traffic of HSPCs and leukocytes.
© 2019 by The American Society of Hematology.
Conflict of interest statement
Conflict-of-interest disclosure: The authors declare no competing financial interests.
Figures
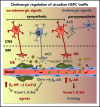
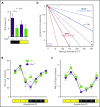
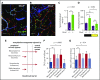
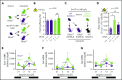
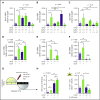
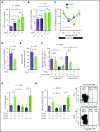
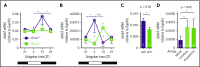
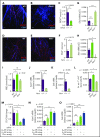
Comment in
-
Timing's everything in light, location, and cells.Blood. 2019 Jan 17;133(3):186-187. doi: 10.1182/blood-2018-11-883462. Blood. 2019. PMID: 30655302 No abstract available.
Similar articles
-
Signals from the sympathetic nervous system regulate hematopoietic stem cell egress from bone marrow.Cell. 2006 Jan 27;124(2):407-21. doi: 10.1016/j.cell.2005.10.041. Cell. 2006. PMID: 16439213
-
The Involvment of Hematopoietic-Specific PLC -β2 in Homing and Engraftment of Hematopoietic Stem/Progenitor Cells.Stem Cell Rev Rep. 2016 Dec;12(6):613-620. doi: 10.1007/s12015-016-9689-x. Stem Cell Rev Rep. 2016. PMID: 27704316 Free PMC article.
-
Endocannabinoids are expressed in bone marrow stromal niches and play a role in interactions of hematopoietic stem and progenitor cells with the bone marrow microenvironment.J Biol Chem. 2010 Nov 12;285(46):35471-8. doi: 10.1074/jbc.M110.144758. Epub 2010 Sep 7. J Biol Chem. 2010. Retraction in: J Biol Chem. 2011 Jul 8;286(27):24534. doi: 10.1074/jbc.a110.144758 PMID: 20826813 Free PMC article. Retracted.
-
The Autonomic Nervous System Pulls the Strings to Coordinate Circadian HSC Functions.Front Immunol. 2020 May 20;11:956. doi: 10.3389/fimmu.2020.00956. eCollection 2020. Front Immunol. 2020. PMID: 32508835 Free PMC article. Review.
-
Circadian rhythms, adrenergic hormones and trafficking of hematopoietic stem cells.Expert Opin Ther Targets. 2010 May;14(5):567-75. doi: 10.1517/14728221003769887. Expert Opin Ther Targets. 2010. PMID: 20350049 Review.
Cited by
-
Cholinergic signals preserve haematopoietic stem cell quiescence during regenerative haematopoiesis.Nat Commun. 2022 Jan 27;13(1):543. doi: 10.1038/s41467-022-28175-1. Nat Commun. 2022. PMID: 35087060 Free PMC article.
-
Circadian Features of Neutrophil Biology.Front Immunol. 2020 Apr 3;11:576. doi: 10.3389/fimmu.2020.00576. eCollection 2020. Front Immunol. 2020. PMID: 32346378 Free PMC article. Review.
-
Dopamine signaling regulates hematopoietic stem and progenitor cell function.Blood. 2021 Nov 25;138(21):2051-2065. doi: 10.1182/blood.2020010419. Blood. 2021. PMID: 34370827 Free PMC article.
-
Megakaryocyte Diversity in Ontogeny, Functions and Cell-Cell Interactions.Front Oncol. 2022 Feb 4;12:840044. doi: 10.3389/fonc.2022.840044. eCollection 2022. Front Oncol. 2022. PMID: 35186768 Free PMC article.
-
In vivo vesicular acetylcholine transporter density in human peripheral organs: an [18F]FEOBV PET/CT study.EJNMMI Res. 2022 Apr 1;12(1):17. doi: 10.1186/s13550-022-00889-9. EJNMMI Res. 2022. PMID: 35362761 Free PMC article.
References
-
- Francis NJ, Landis SC. Cellular and molecular determinants of sympathetic neuron development. Annu Rev Neurosci. 1999;22(1):541-566. - PubMed
-
- Apostolova G, Dechant G. Development of neurotransmitter phenotypes in sympathetic neurons. Auton Neurosci. 2009;151(1):30-38. - PubMed
-
- Cane KN, Anderson CR. Generating diversity: Mechanisms regulating the differentiation of autonomic neuron phenotypes. Auton Neurosci. 2009;151(1):17-29. - PubMed
-
- Yamazaki K, Allen TD. Ultrastructural morphometric study of efferent nerve terminals on murine bone marrow stromal cells, and the recognition of a novel anatomical unit: the “neuro-reticular complex.” Am J Anat. 1990;187(3):261-276. - PubMed
Publication types
MeSH terms
Substances
Grants and funding
LinkOut - more resources
Full Text Sources
Medical
Molecular Biology Databases