Human Organ-Specific Endothelial Cell Heterogeneity
- PMID: 30240741
- PMCID: PMC6147238
- DOI: 10.1016/j.isci.2018.05.003
Human Organ-Specific Endothelial Cell Heterogeneity
Abstract
The endothelium first forms in the blood islands in the extra-embryonic yolk sac and then throughout the embryo to establish circulatory networks that further acquire organ-specific properties during development to support diverse organ functions. Here, we investigated the properties of endothelial cells (ECs), isolated from four human major organs-the heart, lung, liver, and kidneys-in individual fetal tissues at three months' gestation, at gene expression, and at cellular function levels. We showed that organ-specific ECs have distinct expression patterns of gene clusters, which support their specific organ development and functions. These ECs displayed distinct barrier properties, angiogenic potential, and metabolic rate and support specific organ functions. Our findings showed the link between human EC heterogeneity and organ development and can be exploited therapeutically to contribute in organ regeneration, disease modeling, as well as guiding differentiation of tissue-specific ECs from human pluripotent stem cells.
Keywords: Biology of Human Development; Developmental Biology; Stem Cells Research.
Copyright © 2018 The Authors. Published by Elsevier Inc. All rights reserved.
Figures
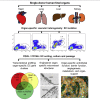
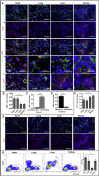
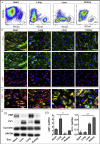
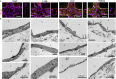
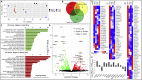
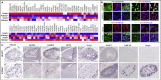

Similar articles
-
Molecular signatures of tissue-specific microvascular endothelial cell heterogeneity in organ maintenance and regeneration.Dev Cell. 2013 Jul 29;26(2):204-19. doi: 10.1016/j.devcel.2013.06.017. Epub 2013 Jul 18. Dev Cell. 2013. PMID: 23871589 Free PMC article.
-
Diversity of Organism-Wide and Organ-Specific Endothelial Cells.Curr Cardiol Rep. 2020 Feb 8;22(4):19. doi: 10.1007/s11886-020-1275-9. Curr Cardiol Rep. 2020. PMID: 32036477 Free PMC article. Review.
-
Wnt signaling mediates acquisition of blood-brain barrier properties in naïve endothelium derived from human pluripotent stem cells.Elife. 2021 Nov 10;10:e70992. doi: 10.7554/eLife.70992. Elife. 2021. PMID: 34755601 Free PMC article.
-
Endothelial heterogeneity across distinct vascular beds during homeostasis and inflammation.Elife. 2020 Jan 16;9:e51413. doi: 10.7554/eLife.51413. Elife. 2020. PMID: 31944177 Free PMC article.
-
Vascularizing organogenesis: Lessons from developmental biology and implications for regenerative medicine.Curr Top Dev Biol. 2019;132:177-220. doi: 10.1016/bs.ctdb.2018.12.012. Epub 2019 Jan 18. Curr Top Dev Biol. 2019. PMID: 30797509 Review.
Cited by
-
Monomeric and oligomeric amyloid-β cause distinct Alzheimer's disease pathophysiological characteristics in astrocytes in human glymphatics-on-chip models.Lab Chip. 2024 Aug 6;24(16):3826-3839. doi: 10.1039/d4lc00287c. Lab Chip. 2024. PMID: 39037244 Free PMC article.
-
Organ-Specific Mechanisms of Transendothelial Neutrophil Migration in the Lung, Liver, Kidney, and Aorta.Front Immunol. 2018 Nov 27;9:2739. doi: 10.3389/fimmu.2018.02739. eCollection 2018. Front Immunol. 2018. PMID: 30538702 Free PMC article. Review.
-
Endothelial cells and organ function: applications and implications of understanding unique and reciprocal remodelling.FEBS J. 2020 Mar;287(6):1088-1100. doi: 10.1111/febs.15143. Epub 2019 Dec 4. FEBS J. 2020. PMID: 31736207 Free PMC article. Review.
-
Angiogenic Function of Human Placental Endothelial Cells in Severe Fetal Growth Restriction Is Not Rescued by Individual Extracellular Matrix Proteins.Cells. 2023 Sep 23;12(19):2339. doi: 10.3390/cells12192339. Cells. 2023. PMID: 37830553 Free PMC article.
-
Gut bioengineering promotes gut repair and pharmaceutical research: a review.J Tissue Eng. 2019 Apr 19;10:2041731419839846. doi: 10.1177/2041731419839846. eCollection 2019 Jan-Dec. J Tissue Eng. 2019. PMID: 31037215 Free PMC article. Review.
References
-
- Adams R.H., Alitalo K. Molecular regulation of angiogenesis and lymphangiogenesis. Nat. Rev. Mol. Cell Biol. 2007;8:464–478. - PubMed
-
- Aird W.C. Phenotypic heterogeneity of the endothelium I. Structure, function, and mechanisms. Circ. Res. 2007;100:158–173. - PubMed
-
- Aird W.C. Phenotypic heterogeneity of the endothelium II. Representative vascular beds. Circ. Res. 2007;100:174–190. - PubMed
Grants and funding
LinkOut - more resources
Full Text Sources
Other Literature Sources
Molecular Biology Databases