Transcriptional and epigenetic profiling of nutrient-deprived cells to identify novel regulators of autophagy
- PMID: 30153076
- PMCID: PMC6287694
- DOI: 10.1080/15548627.2018.1509608
Transcriptional and epigenetic profiling of nutrient-deprived cells to identify novel regulators of autophagy
Abstract
Macroautophagy (hereafter autophagy) is a lysosomal degradation pathway critical for maintaining cellular homeostasis and viability, and is predominantly regarded as a rapid and dynamic cytoplasmic process. To increase our understanding of the transcriptional and epigenetic events associated with autophagy, we performed extensive genome-wide transcriptomic and epigenomic profiling after nutrient deprivation in human autophagy-proficient and autophagy-deficient cells. We observed that nutrient deprivation leads to the transcriptional induction of numerous autophagy-associated genes. These transcriptional changes are reflected at the epigenetic level (H3K4me3, H3K27ac, and H3K56ac) and are independent of autophagic flux. As a proof of principle that this resource can be used to identify novel autophagy regulators, we followed up on one identified target: EGR1 (early growth response 1), which indeed appears to be a central transcriptional regulator of autophagy by affecting autophagy-associated gene expression and autophagic flux. Taken together, these data stress the relevance of transcriptional and epigenetic regulation of autophagy and can be used as a resource to identify (novel) factors involved in autophagy regulation.
Keywords: Autophagy; ChIP-seq; EGR1; RNA-seq; nutrient-deprivation.
Figures
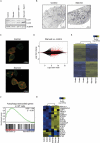
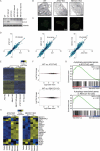
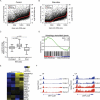
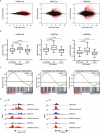
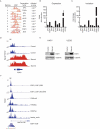
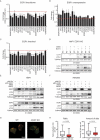
Similar articles
-
Epigenetic regulation of autophagy by histone-modifying enzymes under nutrient stress.Cell Death Differ. 2023 Jun;30(6):1430-1436. doi: 10.1038/s41418-023-01154-9. Epub 2023 Mar 30. Cell Death Differ. 2023. PMID: 36997734 Free PMC article. Review.
-
PHF20 is crucial for epigenetic control of starvation-induced autophagy through enhancer activation.Nucleic Acids Res. 2022 Aug 12;50(14):7856-7872. doi: 10.1093/nar/gkac584. Nucleic Acids Res. 2022. PMID: 35821310 Free PMC article.
-
Transcriptional and Epigenetic Regulation of Autophagy in Plants.Trends Genet. 2020 Sep;36(9):676-688. doi: 10.1016/j.tig.2020.06.013. Epub 2020 Jul 13. Trends Genet. 2020. PMID: 32674948 Review.
-
Transcriptional regulation of mammalian autophagy at a glance.J Cell Sci. 2016 Aug 15;129(16):3059-66. doi: 10.1242/jcs.188920. J Cell Sci. 2016. PMID: 27528206 Review.
-
Systematic Analysis of Human Cells Lacking ATG8 Proteins Uncovers Roles for GABARAPs and the CCZ1/MON1 Regulator C18orf8/RMC1 in Macroautophagic and Selective Autophagic Flux.Mol Cell Biol. 2017 Dec 13;38(1):e00392-17. doi: 10.1128/MCB.00392-17. Print 2018 Jan 1. Mol Cell Biol. 2017. PMID: 29038162 Free PMC article.
Cited by
-
A dual-activity topoisomerase complex promotes both transcriptional activation and repression in response to starvation.Nucleic Acids Res. 2023 Mar 21;51(5):2415-2433. doi: 10.1093/nar/gkad086. Nucleic Acids Res. 2023. PMID: 36794732 Free PMC article.
-
Differences in Leukocyte Transcriptomes of Morbidly Obese Patients With High Output Heart Failure: A Pilot Study.Int J Heart Fail. 2023 Sep 5;5(4):201-212. doi: 10.36628/ijhf.2023.0027. eCollection 2023 Oct. Int J Heart Fail. 2023. PMID: 37937202 Free PMC article.
-
Autophagy related DNA methylation signature predict clinical prognosis and immune microenvironment in low-grade glioma.Transl Cancer Res. 2022 Jul;11(7):2157-2174. doi: 10.21037/tcr-22-310. Transl Cancer Res. 2022. PMID: 35966301 Free PMC article.
-
Epigenetic Regulation of Autophagy Beyond the Cytoplasm: A Review.Front Cell Dev Biol. 2021 Jun 14;9:675599. doi: 10.3389/fcell.2021.675599. eCollection 2021. Front Cell Dev Biol. 2021. PMID: 34195194 Free PMC article. Review.
-
Mitochondrial carrier protein overloading and misfolding induce aggresomes and proteostatic adaptations in the cytosol.Mol Biol Cell. 2019 May 15;30(11):1272-1284. doi: 10.1091/mbc.E19-01-0046. Epub 2019 Mar 20. Mol Biol Cell. 2019. PMID: 30893019 Free PMC article.
References
-
- Mizushima N. Autophagy in Protein and Organelle Turnover. Cold Spring Harb Symp Quant Biol. 2011;76:397–402. - PubMed
Publication types
MeSH terms
Substances
Grants and funding
LinkOut - more resources
Full Text Sources
Other Literature Sources
Molecular Biology Databases
Research Materials