Conservation of coactivator engagement mechanism enables small-molecule allosteric modulators
- PMID: 30127017
- PMCID: PMC6130367
- DOI: 10.1073/pnas.1806202115
Conservation of coactivator engagement mechanism enables small-molecule allosteric modulators
Abstract
Transcriptional coactivators are a molecular recognition marvel because a single domain within these proteins, the activator binding domain or ABD, interacts with multiple compositionally diverse transcriptional activators. Also remarkable is the structural diversity among ABDs, which range from conformationally dynamic helical motifs to those with a stable core such as a β-barrel. A significant objective is to define conserved properties of ABDs that allow them to interact with disparate activator sequences. The ABD of the coactivator Med25 (activator interaction domain or AcID) is unique in that it contains secondary structural elements that are on both ends of the spectrum: helices and loops that display significant conformational mobility and a seven-stranded β-barrel core that is structurally rigid. Using biophysical approaches, we build a mechanistic model of how AcID forms binary and ternary complexes with three distinct activators; despite its static core, Med25 forms short-lived, conformationally mobile, and structurally distinct complexes with each of the cognate partners. Further, ternary complex formation is facilitated by allosteric communication between binding surfaces on opposing faces of the β-barrel. The model emerging suggests that the conformational shifts and cooperative binding is mediated by a flexible substructure comprised of two dynamic helices and flanking loops, indicating a conserved mechanistic model of activator engagement across ABDs. Targeting a region of this substructure with a small-molecule covalent cochaperone modulates ternary complex formation. Our data support a general strategy for the identification of allosteric small-molecule modulators of ABDs, which are key targets for mechanistic studies as well as therapeutic applications.
Keywords: Med25; allosteric modulator; protein–protein interactions; transcriptional coactivator.
Copyright © 2018 the Author(s). Published by PNAS.
Conflict of interest statement
The authors declare no conflict of interest.
Figures
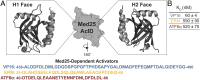
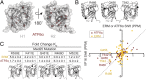
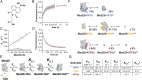
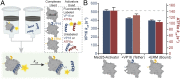
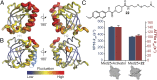
Similar articles
-
A Lipopeptidomimetic of Transcriptional Activation Domains Selectively Disrupts the Coactivator Med25 Protein-Protein Interactions.Angew Chem Int Ed Engl. 2024 May 21;63(21):e202400781. doi: 10.1002/anie.202400781. Epub 2024 Apr 17. Angew Chem Int Ed Engl. 2024. PMID: 38527936 Free PMC article.
-
Unexpected specificity within dynamic transcriptional protein-protein complexes.Proc Natl Acad Sci U S A. 2020 Nov 3;117(44):27346-27353. doi: 10.1073/pnas.2013244117. Epub 2020 Oct 19. Proc Natl Acad Sci U S A. 2020. PMID: 33077600 Free PMC article.
-
Structure and VP16 binding of the Mediator Med25 activator interaction domain.Nat Struct Mol Biol. 2011 Apr;18(4):404-9. doi: 10.1038/nsmb.1997. Epub 2011 Mar 6. Nat Struct Mol Biol. 2011. PMID: 21378965
-
Emerging issues of connexin channels: biophysics fills the gap.Q Rev Biophys. 2001 Aug;34(3):325-472. doi: 10.1017/s0033583501003705. Q Rev Biophys. 2001. PMID: 11838236 Review.
-
The Mediator complex and the role of protein-protein interactions in the gene regulation machinery.Semin Cell Dev Biol. 2020 Mar;99:20-30. doi: 10.1016/j.semcdb.2018.08.006. Epub 2018 Oct 16. Semin Cell Dev Biol. 2020. PMID: 30278226 Review.
Cited by
-
Controlling Intramolecular Interactions in the Design of Selective, High-Affinity Ligands for the CREBBP Bromodomain.J Med Chem. 2021 Jul 22;64(14):10102-10123. doi: 10.1021/acs.jmedchem.1c00348. Epub 2021 Jul 13. J Med Chem. 2021. PMID: 34255515 Free PMC article.
-
Multivalent and Bidirectional Binding of Transcriptional Transactivation Domains to the MED25 Coactivator.Biomolecules. 2020 Aug 19;10(9):1205. doi: 10.3390/biom10091205. Biomolecules. 2020. PMID: 32825095 Free PMC article.
-
Molecular switching in transcription through splicing and proline-isomerization regulates stress responses in plants.Nat Commun. 2024 Jan 18;15(1):592. doi: 10.1038/s41467-024-44859-2. Nat Commun. 2024. PMID: 38238333 Free PMC article.
-
A Lipopeptidomimetic of Transcriptional Activation Domains Selectively Disrupts the Coactivator Med25 Protein-Protein Interactions.Angew Chem Int Ed Engl. 2024 May 21;63(21):e202400781. doi: 10.1002/anie.202400781. Epub 2024 Apr 17. Angew Chem Int Ed Engl. 2024. PMID: 38527936 Free PMC article.
-
Mediator subunit Med15 dictates the conserved "fuzzy" binding mechanism of yeast transcription activators Gal4 and Gcn4.Nat Commun. 2021 Apr 13;12(1):2220. doi: 10.1038/s41467-021-22441-4. Nat Commun. 2021. PMID: 33850123 Free PMC article.
References
Publication types
MeSH terms
Substances
Grants and funding
LinkOut - more resources
Full Text Sources
Other Literature Sources