Heterosubtypic Protections against Human-Infecting Avian Influenza Viruses Correlate to Biased Cross-T-Cell Responses
- PMID: 30087171
- PMCID: PMC6083907
- DOI: 10.1128/mBio.01408-18
Heterosubtypic Protections against Human-Infecting Avian Influenza Viruses Correlate to Biased Cross-T-Cell Responses
Abstract
Against a backdrop of seasonal influenza virus epidemics, emerging avian influenza viruses (AIVs) occasionally jump from birds to humans, posing a public health risk, especially with the recent sharp increase in H7N9 infections. Evaluations of cross-reactive T-cell immunity to seasonal influenza viruses and human-infecting AIVs have been reported previously. However, the roles of influenza A virus-derived epitopes in the cross-reactive T-cell responses and heterosubtypic protections are not well understood; understanding those roles is important for preventing and controlling new emerging AIVs. Here, among the members of a healthy population presumed to have previously been infected by pandemic H1N1 (pH1N1), we found that pH1N1-specific T cells showed cross- but biased reactivity to human-infecting AIVs, i.e., H5N1, H6N1, H7N9, and H9N2, which correlates with distinct protections. Through a T-cell epitope-based phylogenetic analysis, the cellular immunogenic clustering expanded the relevant conclusions to a broader range of virus strains. We defined the potential key conserved epitopes required for cross-protection and revealed the molecular basis for the immunogenic variations. Our study elucidated an overall profile of cross-reactivity to AIVs and provided useful recommendations for broad-spectrum vaccine development.IMPORTANCE We revealed preexisting but biased T-cell reactivity of pH1N1 influenza virus to human-infecting AIVs, which provided distinct protections. The cross-reactive T-cell recognition had a regular pattern that depended on the T-cell epitope matrix revealed via bioinformatics analysis. Our study elucidated an overall profile of cross-reactivity to AIVs and provided useful recommendations for broad-spectrum vaccine development.
Keywords: T-cell responses; avian influenza viruses; cross-reactivity; heterosubtypic protection.
Copyright © 2018 Zhao et al.
Figures
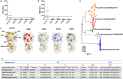
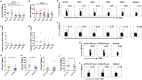
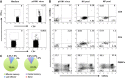
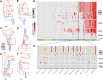
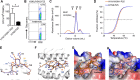
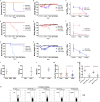
Similar articles
-
Evaluation of in vitro cross-reactivity to avian H5N1 and pandemic H1N1 2009 influenza following prime boost regimens of seasonal influenza vaccination in healthy human subjects: a randomised trial.PLoS One. 2013;8(3):e59674. doi: 10.1371/journal.pone.0059674. Epub 2013 Mar 26. PLoS One. 2013. PMID: 23555741 Free PMC article. Clinical Trial.
-
Neuraminidase-Inhibiting Antibody Titers Correlate with Protection from Heterologous Influenza Virus Strains of the Same Neuraminidase Subtype.J Virol. 2018 Aug 16;92(17):e01006-18. doi: 10.1128/JVI.01006-18. Print 2018 Sep 1. J Virol. 2018. PMID: 29925654 Free PMC article.
-
Cross-Reactive Neuraminidase-Inhibiting Antibodies Elicited by Immunization with Recombinant Neuraminidase Proteins of H5N1 and Pandemic H1N1 Influenza A Viruses.J Virol. 2015 Jul;89(14):7224-34. doi: 10.1128/JVI.00585-15. Epub 2015 May 6. J Virol. 2015. PMID: 25948745 Free PMC article.
-
Immune responses to infection with H5N1 influenza virus.Virus Res. 2013 Dec 5;178(1):44-52. doi: 10.1016/j.virusres.2013.05.011. Epub 2013 Jun 2. Virus Res. 2013. PMID: 23735534 Free PMC article. Review.
-
Avian Influenza Viruses, Inflammation, and CD8(+) T Cell Immunity.Front Immunol. 2016 Mar 1;7:60. doi: 10.3389/fimmu.2016.00060. eCollection 2016. Front Immunol. 2016. PMID: 26973644 Free PMC article. Review.
Cited by
-
Cross-species recognition of SARS-CoV-2 to bat ACE2.Proc Natl Acad Sci U S A. 2021 Jan 5;118(1):e2020216118. doi: 10.1073/pnas.2020216118. Proc Natl Acad Sci U S A. 2021. PMID: 33335073 Free PMC article.
-
N-glycosylation of PD-1 promotes binding of camrelizumab.EMBO Rep. 2020 Dec 3;21(12):e51444. doi: 10.15252/embr.202051444. Epub 2020 Oct 15. EMBO Rep. 2020. PMID: 33063473 Free PMC article.
-
Rapid humoral immune responses are required for recovery from haemorrhagic fever with renal syndrome patients.Emerg Microbes Infect. 2020 Dec;9(1):2303-2314. doi: 10.1080/22221751.2020.1830717. Emerg Microbes Infect. 2020. PMID: 32990499 Free PMC article.
-
Seeing the T cell Immunity of SARS-CoV-2 and SARS-CoV: Believing the Epitope-Oriented Vaccines.Int J Biol Sci. 2023 Aug 6;19(13):4052-4060. doi: 10.7150/ijbs.80468. eCollection 2023. Int J Biol Sci. 2023. PMID: 37705735 Free PMC article. Review.
-
Safety, Immunogenicity, and Protective Efficacy of a Chimeric A/B Live Attenuated Influenza Vaccine in a Mouse Model.Microorganisms. 2021 Jan 27;9(2):259. doi: 10.3390/microorganisms9020259. Microorganisms. 2021. PMID: 33513862 Free PMC article.
References
-
- Gaglani M, Pruszynski J, Murthy K, Clipper L, Robertson A, Reis M, Chung JR, Piedra PA, Avadhanula V, Nowalk MP, Zimmerman RK, Jackson ML, Jackson LA, Petrie JG, Ohmit SE, Monto AS, McLean HQ, Belongia EA, Fry AM, Flannery B. 2016. Influenza vaccine effectiveness against 2009 pandemic influenza A(H1N1) virus differed by vaccine type during 2013–2014 in the United States. J Infect Dis 213:1546–1556. doi:10.1093/infdis/jiv577. - DOI - PMC - PubMed
-
- Van Kerkhove MD, Hirve S, Koukounari A, Mounts AW; H1N1pdm serology working group. 2013. Estimating age-specific cumulative incidence for the 2009 influenza pandemic: a meta-analysis of A(H1N1)pdm09 serological studies from 19 countries. Influenza Other Respir Viruses 7:872–886. doi:10.1111/irv.12074. - DOI - PMC - PubMed
Publication types
MeSH terms
Substances
LinkOut - more resources
Full Text Sources
Other Literature Sources
Medical