Multilevel effects of light on ribosome dynamics in chloroplasts program genome-wide and psbA-specific changes in translation
- PMID: 30080854
- PMCID: PMC6095610
- DOI: 10.1371/journal.pgen.1007555
Multilevel effects of light on ribosome dynamics in chloroplasts program genome-wide and psbA-specific changes in translation
Erratum in
-
Correction: Multilevel effects of light on ribosome dynamics in chloroplasts program genome-wide and psbA-specific changes in translation.PLoS Genet. 2019 Jan 3;15(1):e1007907. doi: 10.1371/journal.pgen.1007907. eCollection 2019 Jan. PLoS Genet. 2019. PMID: 30605477 Free PMC article.
Abstract
Plants and algae adapt to fluctuating light conditions to optimize photosynthesis, minimize photodamage, and prioritize energy investments. Changes in the translation of chloroplast mRNAs are known to contribute to these adaptations, but the scope and magnitude of these responses are unclear. To clarify the phenomenology, we used ribosome profiling to analyze chloroplast translation in maize seedlings following dark-to-light and light-to-dark shifts. The results resolved several layers of regulation. (i) The psbA mRNA exhibits a dramatic gain of ribosomes within minutes after shifting plants to the light and reverts to low ribosome occupancy within one hour in the dark, correlating with the need to replace damaged PsbA in Photosystem II. (ii) Ribosome occupancy on all other chloroplast mRNAs remains similar to that at midday even after 12 hours in the dark. (iii) Analysis of ribosome dynamics in the presence of lincomycin revealed a global decrease in the translation elongation rate shortly after shifting plants to the dark. The pausing of chloroplast ribosomes at specific sites changed very little during these light-shift regimes. A similar but less comprehensive analysis in Arabidopsis gave similar results excepting a trend toward reduced ribosome occupancy at the end of the night. Our results show that all chloroplast mRNAs except psbA maintain similar ribosome occupancy following short-term light shifts, but are nonetheless translated at higher rates in the light due to a plastome-wide increase in elongation rate. A light-induced recruitment of ribosomes to psbA mRNA is superimposed on this global response, producing a rapid and massive increase in PsbA synthesis. These findings highlight the unique translational response of psbA in mature chloroplasts, clarify which steps in psbA translation are light-regulated in the context of Photosystem II repair, and provide a foundation on which to explore mechanisms underlying the psbA-specific and global effects of light on chloroplast translation.
Conflict of interest statement
The authors have declared that no competing interests exist.
Figures
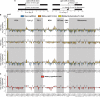
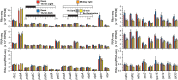
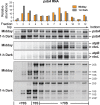
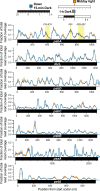
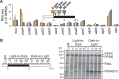
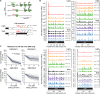
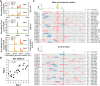
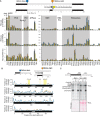
Similar articles
-
Light-induced psbA translation in plants is triggered by photosystem II damage via an assembly-linked autoregulatory circuit.Proc Natl Acad Sci U S A. 2020 Sep 1;117(35):21775-21784. doi: 10.1073/pnas.2007833117. Epub 2020 Aug 18. Proc Natl Acad Sci U S A. 2020. PMID: 32817480 Free PMC article.
-
Exploring the proteome associated with the mRNA encoding the D1 reaction center protein of Photosystem II in plant chloroplasts.Plant J. 2020 Apr;102(2):369-382. doi: 10.1111/tpj.14629. Epub 2020 Jan 16. Plant J. 2020. PMID: 31793101
-
Ribosome-binding sites on chloroplast rbcL and psbA mRNAs and light-induced initiation of D1 translation.Plant Mol Biol. 1994 Jun;25(3):437-48. doi: 10.1007/BF00043872. Plant Mol Biol. 1994. PMID: 8049369
-
Chloroplast translation regulation.Photosynth Res. 2007 Nov-Dec;94(2-3):359-74. doi: 10.1007/s11120-007-9183-z. Epub 2007 Jul 28. Photosynth Res. 2007. PMID: 17661159 Review.
-
Strategies for psbA gene expression in cyanobacteria, green algae and higher plants: from transcription to PSII repair.Biochim Biophys Acta. 2012 Jan;1817(1):247-57. doi: 10.1016/j.bbabio.2011.04.011. Epub 2011 May 2. Biochim Biophys Acta. 2012. PMID: 21565160 Review.
Cited by
-
Translational Regulation of Duplicated Gene Expression Evolution in Allopolyploid Cotton.Genes (Basel). 2024 Aug 27;15(9):1130. doi: 10.3390/genes15091130. Genes (Basel). 2024. PMID: 39336721 Free PMC article.
-
Limited Responsiveness of Chloroplast Gene Expression during Acclimation to High Light in Tobacco.Plant Physiol. 2020 Jan;182(1):424-435. doi: 10.1104/pp.19.00953. Epub 2019 Oct 21. Plant Physiol. 2020. PMID: 31636102 Free PMC article.
-
Correction: Multilevel effects of light on ribosome dynamics in chloroplasts program genome-wide and psbA-specific changes in translation.PLoS Genet. 2019 Jan 3;15(1):e1007907. doi: 10.1371/journal.pgen.1007907. eCollection 2019 Jan. PLoS Genet. 2019. PMID: 30605477 Free PMC article.
-
Arabidopsis homeobox-leucine zipper transcription factor BRASSINOSTEROID-RELATED HOMEOBOX 3 regulates leaf greenness by suppressing BR signaling.Plant Biotechnol (Tokyo). 2022 Jun 25;39(2):209-214. doi: 10.5511/plantbiotechnology.22.0128a. Plant Biotechnol (Tokyo). 2022. PMID: 35937537 Free PMC article.
-
Experimental approaches to studying translation in plant semi-autonomous organelles.J Exp Bot. 2024 Sep 11;75(17):5175-5187. doi: 10.1093/jxb/erae151. J Exp Bot. 2024. PMID: 38592734 Free PMC article. Review.
References
Publication types
MeSH terms
Substances
Grants and funding
LinkOut - more resources
Full Text Sources
Other Literature Sources
Molecular Biology Databases