High-Throughput Screening Enhances Kidney Organoid Differentiation from Human Pluripotent Stem Cells and Enables Automated Multidimensional Phenotyping
- PMID: 29779890
- PMCID: PMC5984728
- DOI: 10.1016/j.stem.2018.04.022
High-Throughput Screening Enhances Kidney Organoid Differentiation from Human Pluripotent Stem Cells and Enables Automated Multidimensional Phenotyping
Abstract
Organoids derived from human pluripotent stem cells are a potentially powerful tool for high-throughput screening (HTS), but the complexity of organoid cultures poses a significant challenge for miniaturization and automation. Here, we present a fully automated, HTS-compatible platform for enhanced differentiation and phenotyping of human kidney organoids. The entire 21-day protocol, from plating to differentiation to analysis, can be performed automatically by liquid-handling robots, or alternatively by manual pipetting. High-content imaging analysis reveals both dose-dependent and threshold effects during organoid differentiation. Immunofluorescence and single-cell RNA sequencing identify previously undetected parietal, interstitial, and partially differentiated compartments within organoids and define conditions that greatly expand the vascular endothelium. Chemical modulation of toxicity and disease phenotypes can be quantified for safety and efficacy prediction. Screening in gene-edited organoids in this system reveals an unexpected role for myosin in polycystic kidney disease. Organoids in HTS formats thus establish an attractive platform for multidimensional phenotypic screening.
Copyright © 2018 Elsevier Inc. All rights reserved.
Figures
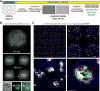
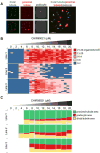
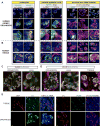
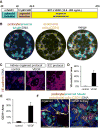
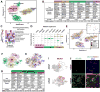
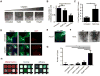
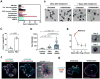
Similar articles
-
A fully automated high-throughput workflow for 3D-based chemical screening in human midbrain organoids.Elife. 2020 Nov 3;9:e52904. doi: 10.7554/eLife.52904. Elife. 2020. PMID: 33138918 Free PMC article.
-
Pluripotent stem cell-derived kidney organoids: An in vivo-like in vitro technology.Eur J Pharmacol. 2016 Nov 5;790:12-20. doi: 10.1016/j.ejphar.2016.06.059. Epub 2016 Jul 1. Eur J Pharmacol. 2016. PMID: 27375081 Review.
-
Development of an automated 3D high content cell screening platform for organoid phenotyping.SLAS Discov. 2024 Oct;29(7):100182. doi: 10.1016/j.slasd.2024.100182. Epub 2024 Sep 6. SLAS Discov. 2024. PMID: 39245180
-
Generation of Human PSC-Derived Kidney Organoids with Patterned Nephron Segments and a De Novo Vascular Network.Cell Stem Cell. 2019 Sep 5;25(3):373-387.e9. doi: 10.1016/j.stem.2019.06.009. Epub 2019 Jul 11. Cell Stem Cell. 2019. PMID: 31303547 Free PMC article.
-
Single Cell Sequencing and Kidney Organoids Generated from Pluripotent Stem Cells.Clin J Am Soc Nephrol. 2020 Apr 7;15(4):550-556. doi: 10.2215/CJN.07470619. Epub 2020 Jan 28. Clin J Am Soc Nephrol. 2020. PMID: 31992574 Free PMC article. Review.
Cited by
-
Scalable production of uniform and mature organoids in a 3D geometrically-engineered permeable membrane.Nat Commun. 2024 Oct 31;15(1):9420. doi: 10.1038/s41467-024-53073-z. Nat Commun. 2024. PMID: 39482314 Free PMC article.
-
Organoids in the oral and maxillofacial region: present and future.Int J Oral Sci. 2024 Nov 1;16(1):61. doi: 10.1038/s41368-024-00324-w. Int J Oral Sci. 2024. PMID: 39482304 Free PMC article. Review.
-
Human midbrain organoids: a powerful tool for advanced Parkinson's disease modeling and therapy exploration.NPJ Parkinsons Dis. 2024 Oct 20;10(1):189. doi: 10.1038/s41531-024-00799-8. NPJ Parkinsons Dis. 2024. PMID: 39428415 Free PMC article. Review.
-
Organoid modeling meets cancers of female reproductive tract.Cell Death Discov. 2024 Sep 27;10(1):410. doi: 10.1038/s41420-024-02186-x. Cell Death Discov. 2024. PMID: 39333482 Free PMC article. Review.
-
Light-field tomographic fluorescence lifetime imaging microscopy.Proc Natl Acad Sci U S A. 2024 Oct;121(40):e2402556121. doi: 10.1073/pnas.2402556121. Epub 2024 Sep 25. Proc Natl Acad Sci U S A. 2024. PMID: 39320920
References
-
- Astashkina AI, Mann BK, Prestwich GD, Grainger DW. A 3-D organoid kidney culture model engineered for high-throughput nephrotoxicity assays. Biomaterials. 2012;33:4700–4711. - PubMed
-
- Chen S, Borowiak M, Fox JL, Maehr R, Osafune K, Davidow L, Lam K, Peng LF, Schreiber SL, Rubin LL, et al. A small molecule that directs differentiation of human ESCs into the pancreatic lineage. Nature Chemical Biology. 2009;5:258–265. - PubMed
MeSH terms
Grants and funding
LinkOut - more resources
Full Text Sources
Other Literature Sources
Miscellaneous