Mechanotransduction via the LINC complex regulates DNA replication in myonuclei
- PMID: 29650775
- PMCID: PMC5987719
- DOI: 10.1083/jcb.201708137
Mechanotransduction via the LINC complex regulates DNA replication in myonuclei
Abstract
Nuclear mechanotransduction has been implicated in the control of chromatin organization; however, its impact on functional contractile myofibers is unclear. We found that deleting components of the linker of nucleoskeleton and cytoskeleton (LINC) complex in Drosophila melanogaster larval muscles abolishes the controlled and synchronized DNA endoreplication, typical of nuclei across myofibers, resulting in increased and variable DNA content in myonuclei of individual myofibers. Moreover, perturbation of LINC-independent mechanical input after knockdown of β-Integrin in larval muscles similarly led to increased DNA content in myonuclei. Genome-wide RNA-polymerase II occupancy analysis in myofibers of the LINC mutant klar indicated an altered binding profile, including a significant decrease in the chromatin regulator barrier-to-autointegration factor (BAF) and the contractile regulator Troponin C. Importantly, muscle-specific knockdown of BAF led to increased DNA content in myonuclei, phenocopying the LINC mutant phenotype. We propose that mechanical stimuli transmitted via the LINC complex act via BAF to regulate synchronized cell-cycle progression of myonuclei across single myofibers.
© 2018 Wang et al.
Figures
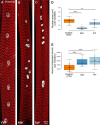
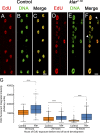
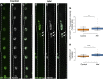
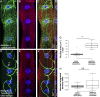
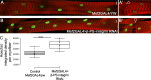
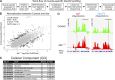
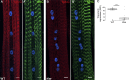
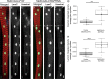
Similar articles
-
Recruitment of BAF to the nuclear envelope couples the LINC complex to endoreplication.Development. 2020 Dec 13;147(23):dev191304. doi: 10.1242/dev.191304. Development. 2020. PMID: 33168584 Free PMC article.
-
A minimal constraint device for imaging nuclei in live Drosophila contractile larval muscles reveals novel nuclear mechanical dynamics.Lab Chip. 2020 Jun 21;20(12):2100-2112. doi: 10.1039/d0lc00214c. Epub 2020 May 20. Lab Chip. 2020. PMID: 32432302
-
Outer nuclear membrane protein Kuduk modulates the LINC complex and nuclear envelope architecture.J Cell Biol. 2017 Sep 4;216(9):2827-2841. doi: 10.1083/jcb.201606043. Epub 2017 Jul 17. J Cell Biol. 2017. PMID: 28716842 Free PMC article.
-
LINC complex proteins in development and disease.Curr Top Dev Biol. 2014;109:287-321. doi: 10.1016/B978-0-12-397920-9.00004-4. Curr Top Dev Biol. 2014. PMID: 24947240 Review.
-
Dynamic regulation of LINC complex composition and function across tissues and contexts.FEBS Lett. 2023 Nov;597(22):2823-2832. doi: 10.1002/1873-3468.14757. Epub 2023 Oct 24. FEBS Lett. 2023. PMID: 37846646 Review.
Cited by
-
Regulation of Nuclear Mechanics and the Impact on DNA Damage.Int J Mol Sci. 2021 Mar 20;22(6):3178. doi: 10.3390/ijms22063178. Int J Mol Sci. 2021. PMID: 33804722 Free PMC article. Review.
-
Microtubule Organization in Striated Muscle Cells.Cells. 2020 Jun 3;9(6):1395. doi: 10.3390/cells9061395. Cells. 2020. PMID: 32503326 Free PMC article. Review.
-
The LINC Complex Inhibits Excessive Chromatin Repression.Cells. 2023 Mar 18;12(6):932. doi: 10.3390/cells12060932. Cells. 2023. PMID: 36980273 Free PMC article.
-
Nesprins are mechanotransducers that discriminate epithelial-mesenchymal transition programs.J Cell Biol. 2020 Oct 5;219(10):e201908036. doi: 10.1083/jcb.201908036. J Cell Biol. 2020. PMID: 32790861 Free PMC article.
-
Centrosomal and Non-Centrosomal Microtubule-Organizing Centers (MTOCs) in Drosophila melanogaster.Cells. 2018 Aug 28;7(9):121. doi: 10.3390/cells7090121. Cells. 2018. PMID: 30154378 Free PMC article. Review.
References
-
- Attali R., Warwar N., Israel A., Gurt I., McNally E., Puckelwartz M., Glick B., Nevo Y., Ben-Neriah Z., and Melki J.. 2009. Mutation of SYNE-1, encoding an essential component of the nuclear lamina, is responsible for autosomal recessive arthrogryposis. Hum. Mol. Genet. 18:3462–3469. 10.1093/hmg/ddp290 - DOI - PubMed
Publication types
MeSH terms
Substances
LinkOut - more resources
Full Text Sources
Other Literature Sources
Molecular Biology Databases