Quantifying Local Molecular Tension Using Intercalated DNA Fluorescence
- PMID: 29473755
- PMCID: PMC6023266
- DOI: 10.1021/acs.nanolett.7b04842
Quantifying Local Molecular Tension Using Intercalated DNA Fluorescence
Abstract
The ability to measure mechanics and forces in biological nanostructures, such as DNA, proteins and cells, is of great importance as a means to analyze biomolecular systems. However, current force detection methods often require specialized instrumentation. Here, we present a novel and versatile method to quantify tension in molecular systems locally and in real time, using intercalated DNA fluorescence. This approach can report forces over a range of at least ∼0.5-65 pN with a resolution of 1-3 pN, using commercially available intercalating dyes and a general-purpose fluorescence microscope. We demonstrate that the method can be easily implemented to report double-stranded (ds)DNA tension in any single-molecule assay that is compatible with fluorescence microscopy. This is particularly useful for multiplexed techniques, where measuring applied force in parallel is technically challenging. Moreover, tension measurements based on local dye binding offer the unique opportunity to determine how an applied force is distributed locally within biomolecular structures. Exploiting this, we apply our method to quantify the position-dependent force profile along the length of flow-stretched DNA and reveal that stretched and entwined DNA molecules-mimicking catenated DNA structures in vivo-display transient DNA-DNA interactions. The method reported here has obvious and broad applications for the study of DNA and DNA-protein interactions. Additionally, we propose that it could be employed to measure forces in any system to which dsDNA can be tethered, for applications including protein unfolding, chromosome mechanics, cell motility, and DNA nanomachines.
Keywords: DNA; fluorescence microscopy; force sensor; intercalators; molecular tension.
Conflict of interest statement
The authors declare the following competing financial interest(s): The combined optical tweezers and fluorescence technologies and methods used in this article are patented and licensed to LUMICKS B.V., in which I.H., E.J.G.P., and G.J.L.W. have a financial interest.
Figures
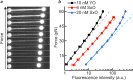
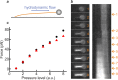
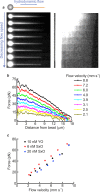
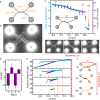
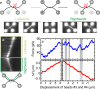
Similar articles
-
Force spectroscopy and fluorescence microscopy of dsDNA-YOYO-1 complexes: implications for the structure of dsDNA in the overstretching region.Nucleic Acids Res. 2010 Jun;38(10):3423-31. doi: 10.1093/nar/gkq034. Epub 2010 Feb 2. Nucleic Acids Res. 2010. PMID: 20129944 Free PMC article.
-
Understanding enhanced mechanical stability of DNA in the presence of intercalated anticancer drug: Implications for DNA associated processes.J Chem Phys. 2019 Oct 28;151(16):164902. doi: 10.1063/1.5117163. J Chem Phys. 2019. PMID: 31675856
-
Direct measurement of conformational changes on DNA molecule intercalating with a fluorescence dye in an electrophoretic buffer solution by means of atomic force microscopy.Electrophoresis. 2001 Oct;22(16):3357-64. doi: 10.1002/1522-2683(200109)22:16<3357::AID-ELPS3357>3.0.CO;2-C. Electrophoresis. 2001. PMID: 11669510
-
Molecular Tension Probes for Imaging Forces at the Cell Surface.Acc Chem Res. 2017 Dec 19;50(12):2915-2924. doi: 10.1021/acs.accounts.7b00305. Epub 2017 Nov 21. Acc Chem Res. 2017. PMID: 29160067 Free PMC article. Review.
-
Advancing Wireframe DNA Nanostructures Using Single-Molecule Fluorescence Microscopy Techniques.Acc Chem Res. 2019 Nov 19;52(11):3199-3210. doi: 10.1021/acs.accounts.9b00424. Epub 2019 Nov 1. Acc Chem Res. 2019. PMID: 31675207 Review.
Cited by
-
Unravelling the mechanisms of Type 1A topoisomerases using single-molecule approaches.Nucleic Acids Res. 2021 Jun 4;49(10):5470-5492. doi: 10.1093/nar/gkab239. Nucleic Acids Res. 2021. PMID: 33963870 Free PMC article. Review.
-
Supercoiling-dependent DNA binding: quantitative modeling and applications to bulk and single-molecule experiments.Nucleic Acids Res. 2024 Jan 11;52(1):59-72. doi: 10.1093/nar/gkad1055. Nucleic Acids Res. 2024. PMID: 38000393 Free PMC article.
-
A Tour de Force on the Double Helix: Exploiting DNA Mechanics To Study DNA-Based Molecular Machines.Biochemistry. 2019 Nov 26;58(47):4667-4676. doi: 10.1021/acs.biochem.9b00346. Epub 2019 Jun 28. Biochemistry. 2019. PMID: 31251042 Free PMC article. Review.
-
Molecular structure, DNA binding mode, photophysical properties and recommendations for use of SYBR Gold.Nucleic Acids Res. 2021 May 21;49(9):5143-5158. doi: 10.1093/nar/gkab265. Nucleic Acids Res. 2021. PMID: 33905507 Free PMC article.
-
Skeletal Muscle Atrophy in Simulated Microgravity Might Be Triggered by Immune-Related microRNAs.Front Physiol. 2019 Jan 10;9:1926. doi: 10.3389/fphys.2018.01926. eCollection 2018. Front Physiol. 2019. PMID: 30687129 Free PMC article.
References
Publication types
MeSH terms
Substances
LinkOut - more resources
Full Text Sources
Other Literature Sources