A single N-terminal phosphomimic disrupts TDP-43 polymerization, phase separation, and RNA splicing
- PMID: 29438978
- PMCID: PMC5830921
- DOI: 10.15252/embj.201797452
A single N-terminal phosphomimic disrupts TDP-43 polymerization, phase separation, and RNA splicing
Abstract
TDP-43 is an RNA-binding protein active in splicing that concentrates into membraneless ribonucleoprotein granules and forms aggregates in amyotrophic lateral sclerosis (ALS) and Alzheimer's disease. Although best known for its predominantly disordered C-terminal domain which mediates ALS inclusions, TDP-43 has a globular N-terminal domain (NTD). Here, we show that TDP-43 NTD assembles into head-to-tail linear chains and that phosphomimetic substitution at S48 disrupts TDP-43 polymeric assembly, discourages liquid-liquid phase separation (LLPS) in vitro, fluidizes liquid-liquid phase separated nuclear TDP-43 reporter constructs in cells, and disrupts RNA splicing activity. Finally, we present the solution NMR structure of a head-to-tail NTD dimer comprised of two engineered variants that allow saturation of the native polymerization interface while disrupting higher-order polymerization. These data provide structural detail for the established mechanistic role of the well-folded TDP-43 NTD in splicing and link this function to LLPS. In addition, the fusion-tag solubilized, recombinant form of TDP-43 full-length protein developed here will enable future phase separation and in vitro biochemical assays on TDP-43 function and interactions that have been hampered in the past by TDP-43 aggregation.
Keywords: RNA splicing; RNP granule; amyotrophic lateral sclerosis; protein–protein interaction; solution NMR spectroscopy.
© 2018 The Authors. Published under the terms of the CC BY 4.0 license.
Figures
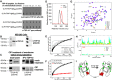
- A
Peptides composed of TDP43 (40–53), with and without phosphorylated Ser48, were serially diluted and spotted to nitrocellulose membranes. Polyclonal antibody (α‐TDP‐43 pSer48) specific to the phosphorylated peptide was used in the top panel showing specificity for pS48, and α‐TDP‐43 “pan antibody” recognizing the same peptide irrespective of phosphorylation was used in the bottom panel.
- B
The α‐TDP‐43 pSer48 antibody and commercial TDP‐43 antibody used in Western blots of HEK293T cell lysates both show reactivity at ˜43 kDa, consistent with TDP‐43 SDS–PAGE migration.
- C
Standard Western blotting was performed on HEK293T cell lysates that had been transferred onto nitrocellulose membranes, except calf intestinal phosphatase (CIP, bottom) or a mock treatment (top) was used to treat the membranes prior to immunoprobing with α‐TDP‐43 (pSer48). Whole HEK293T cell lysates were used in the left panel. In the right panel, TDP‐43 was first immunoprecipitated using commercial α‐TDP‐43 antibody prior to Western blotting.
- D
Gel filtration chromatogram of 200 μM wild‐type (black) and S48E (red) TDP‐43 NTD. The shorter retention time and skewed profile of wild‐type NTD is consistent with self‐assembly. The single‐point variant S48E results in a symmetric peak at longer retention time, consistent with predominantly monomer.
- E
CG‐MALS derived mass average as a function of increasing TDP‐43 NTD concentration data are fit to an isodesmic self‐association model (bold black line) with K D ˜ 95 μM. Fits for dimer, trimer, tetramer, and pentamer models are poor (dashed lines), shown for comparison.
- F
CG‐MALS data for wild‐type are effectively the same at 150 mM (black, repeated from E for clarity) and 300 mM (gray) NaCl. S48E at 150 mM NaCl (red) shows dramatically disrupted assembly with K D ˜ 2,000 μM.
- G, H
The concentration‐dependent chemical shift deviations of 1H‐15N HSQC are large for wild‐type and small for S48E TDP‐43 NTD, consistent with disrupted binding. The CSDs are measured for 200 μM (cyan), 100 μM (green), 40 μM (yellow), and 20 μM (orange) WT compared to a monomeric control: 5 μM. For S48E, only 200 and 100 μM are shown.
- I
The chemical shift deviations (at 100 μM with a cutoff of 0.02 ppm, shown in green) map to two different sides of TDP‐43 NTD (PDB 2N4P), supporting a view that TDP‐43 can assemble into linear chains via multiple interfaces. S48 is highlighted with red spheres.
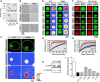
- A
Turbidity of 2.5 μM wild‐type (WT) and S48E TDP‐43‐MBP after 60 min (top) and 120 min (bottom) of incubation with TEV protease is consistent with phase separation at low salt concentration for the wild‐type but phase separation is absent for S48E.
- B
Differential interference contrast micrographs of 2.5 μM full‐length TDP‐43 MBP in 150 mM NaCl (top panel) after 60 and 120 min of incubation with TEV protease. WT shows phase separation, but S48E does not until the concentration is raised (HC).
- C
Wild‐type (WT) and variant (S48E) TDP‐43RRM‐GFP reporters form spherical, micron‐sized nuclear droplets after overnight expression in 293T cells. Nuclei are outlined in white in representative raw images, and heat map representations of the signal intensities measured with standard and sensitive detector settings are provided below to highlight the differences in the nuclear TDP‐43RRM‐GFP reporter signal.
- D
Immunoblot showing total expression levels of WT and S48E reporters in 293T cells. See Appendix Fig S2C for full immunoblots with molecular weight markers.
- E
Representative time‐dependent fluorescence recovery after half‐droplet bleaching shows that S48E enhances intra‐phase diffusion dynamics (decreases viscosity) of TDP‐43 reporter particles.
- F
The phosphomimetic S48E mutation has a dominant, fluidizing effect on the liquid dynamics of composite droplets, as revealed by half‐bleach experiments of composite TDP‐43 droplets formed by co‐expression of wild‐type TDP‐43RRM‐mCherry (red fluorescent, mCh) and the WT (left, blue type) or S48E (right, red type) TDP43RRM‐GFP variants (green fluorescent, GFP) in 293T cells.
- G, H
Quantification of fluorescence recovery after half‐droplet bleaching of (G) GFP wild‐type (black curve) and GFP S48E (red curve) or (H) mixtures of mCherry WT (black squares) plus GFP wild‐type (blue circles) or mixtures of mCherry wild‐type (inverted triangles) plus GFP S48E (red triangles). Error bars indicate s.d. of 20 measured particles from two biological replicates.
- I
Relative splicing activity of CFTR exon 9 minigene reporter in control (N), TDP‐43 siRNA knock‐down (T) HeLa cells, RNA‐binding‐deficient mutant of F147/149L and TDP‐43 NTD variants was calculated as the ratio of percent exon inclusion relative to WT. Levels of exon inclusion using the CFTR exon 9 minigene reporter were quantified as percent of exon inclusion from Appendix Fig S2G. RNAi‐resistant wild‐type TDP‐43 (WT) and mutants were expressed in siRNA‐treated cells. Error bars indicate s.d., n ≥ 4.
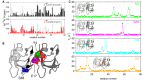
Chemical shift deviations compared to 10 μM alone measured for a mixture of 10 μM 15N wild‐type (WT) and 90 μM S48E (upper, black) identifies the C‐terminal interface, while 10 μM 15N S48E and 90 μM WT (lower, red) TDP‐43 NTD identifies the N‐terminal interface. The gray bars represent the CSDs of 100 μM wild‐type alone compared to 5 μM wild‐type (repeated from Fig 1E).
CSDs with cutoffs of 0.02 ppm (black) and 0.01 ppm (red) mapped on a head‐to‐tail dimer model created from NTD monomer structure (2N4P). The interface residues Y4 (green), E17 (magenta) and S48 (blue) are depicted as spheres.
1HN PREs arising from a mixture of TDP‐43 NTD C39S/C50S and TDP‐43 NTD with a MTSL spin label at position S2C (green), S29C (magenta), C39 (cyan), or C50 (orange) provide structural constraints for a low‐resolution dimer model (Inset). PREs with cutoff of 10 s−1 mapped to the structure of the TDP‐43 NTD.
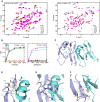
- A
NMR HSQC spectra of 100 μM 15N‐labeled S48E as a function of increasing unlabeled Y4R (right) and 100 μM 15N‐labeled Y4R as a function of increasing unlabeled S48E (left) show resonances in the interface disappear due to exchange between monomeric and complex states, as observed in wild‐type. However, peaks reappear after saturation of the complex (arrows), enabling observation of very large saturated chemical shift deviations consistent with a distinct chemical environment at the interface formed upon dimerization.
- B
Chemical shift mapping of Y4R and S48E TDP‐43 NTD illustrates the saturation of selected resonances of TDP‐43 NTD dimer: S2 (purple square) and T32 (green circle) in one interface, G40 (cyan diamond) and G53 (magenta triangle) in the other, and G69 (orange x) in neither interface.
- C–F
The dimer structure (C) solved for the complex of Y4R (blue) and S48E (cyan) (PDB 6B1G) where panels (D–F) represent the zoom‐in views of the interface. Images of the representative regions highlight intermolecular NOEs shown as yellow dashed lines. The complete NOEs are shown in Appendix Fig S5C.
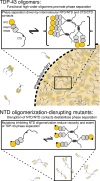
Similar articles
-
Point mutations in the N-terminal domain of transactive response DNA-binding protein 43 kDa (TDP-43) compromise its stability, dimerization, and functions.J Biol Chem. 2017 Jul 14;292(28):11992-12006. doi: 10.1074/jbc.M117.775965. Epub 2017 May 31. J Biol Chem. 2017. PMID: 28566288 Free PMC article.
-
The physical forces mediating self-association and phase-separation in the C-terminal domain of TDP-43.Biochim Biophys Acta Proteins Proteom. 2018 Feb;1866(2):214-223. doi: 10.1016/j.bbapap.2017.10.001. Epub 2017 Oct 4. Biochim Biophys Acta Proteins Proteom. 2018. PMID: 28988034
-
ALS Mutations Disrupt Phase Separation Mediated by α-Helical Structure in the TDP-43 Low-Complexity C-Terminal Domain.Structure. 2016 Sep 6;24(9):1537-49. doi: 10.1016/j.str.2016.07.007. Epub 2016 Aug 18. Structure. 2016. PMID: 27545621 Free PMC article.
-
Role and therapeutic potential of liquid-liquid phase separation in amyotrophic lateral sclerosis.J Mol Cell Biol. 2021 Apr 10;13(1):15-28. doi: 10.1093/jmcb/mjaa049. J Mol Cell Biol. 2021. PMID: 32976566 Free PMC article. Review.
-
Phase to Phase with TDP-43.Biochemistry. 2017 Feb 14;56(6):809-823. doi: 10.1021/acs.biochem.6b01088. Epub 2017 Jan 30. Biochemistry. 2017. PMID: 28112502 Review.
Cited by
-
An Allosteric Modulator of RNA Binding Targeting the N-Terminal Domain of TDP-43 Yields Neuroprotective Properties.ACS Chem Biol. 2020 Nov 20;15(11):2854-2859. doi: 10.1021/acschembio.0c00494. Epub 2020 Oct 12. ACS Chem Biol. 2020. PMID: 33044808 Free PMC article.
-
Recombinant Full-Length TDP-43 Oligomers Retain Their Ability to Bind RNAs, Are Not Toxic, and Do Not Seed TDP-43 Aggregation in Vitro.ACS Chem Neurosci. 2024 Jan 3;15(1):193-204. doi: 10.1021/acschemneuro.3c00691. Epub 2023 Dec 20. ACS Chem Neurosci. 2024. PMID: 38116987 Free PMC article.
-
Cell cycle-specific phase separation regulated by protein charge blockiness.Nat Cell Biol. 2022 May;24(5):625-632. doi: 10.1038/s41556-022-00903-1. Epub 2022 May 5. Nat Cell Biol. 2022. PMID: 35513709 Free PMC article.
-
Physical Chemistry of Cellular Liquid-Phase Separation.Chemistry. 2019 Apr 17;25(22):5600-5610. doi: 10.1002/chem.201805093. Epub 2019 Feb 7. Chemistry. 2019. PMID: 30589142 Free PMC article. Review.
-
RNA Droplets.Annu Rev Biophys. 2020 May 6;49:247-265. doi: 10.1146/annurev-biophys-052118-115508. Epub 2020 Feb 10. Annu Rev Biophys. 2020. PMID: 32040349 Free PMC article. Review.
References
-
- Afroz T, Hock EM, Ernst P, Foglieni C, Jambeau M, Gilhespy LAB, Laferriere F, Maniecka Z, Pluckthun A, Mittl P, Paganetti P, Allain FHT, Polymenidou M (2017) Functional and dynamic polymerization of the ALS‐linked protein TDP‐43 antagonizes its pathologic aggregation. Nat Commun 8: 45 - PMC - PubMed
-
- Alami NH, Smith RB, Carrasco MA, Williams LA, Winborn CS, Han SS, Kiskinis E, Winborn B, Freibaum BD, Kanagaraj A, Clare AJ, Badders NM, Bilican B, Chaum E, Chandran S, Shaw CE, Eggan KC, Maniatis T, Taylor JP (2014) Axonal transport of TDP‐43 mRNA granules is impaired by ALS‐causing mutations. Neuron 81: 536–543 - PMC - PubMed
-
- Arai T, Hasegawa M, Akiyama H, Ikeda K, Nonaka T, Mori H, Mann D, Tsuchiya K, Yoshida M, Hashizume Y, Oda T (2006) TDP‐43 is a component of ubiquitin‐positive tau‐negative inclusions in frontotemporal lobar degeneration and amyotrophic lateral sclerosis. Biochem Biophys Res Commun 351: 602–611 - PubMed
-
- Arnold ES, Ling SC, Huelga SC, Lagier‐Tourenne C, Polymenidou M, Ditsworth D, Kordasiewicz HB, McAlonis‐Downes M, Platoshyn O, Parone PA, Da Cruz S, Clutario KM, Swing D, Tessarollo L, Marsala M, Shaw CE, Yeo GW, Cleveland DW (2013) ALS‐linked TDP‐43 mutations produce aberrant RNA splicing and adult‐onset motor neuron disease without aggregation or loss of nuclear TDP‐43. Proc Natl Acad Sci USA 110: E736–E745 - PMC - PubMed
Publication types
MeSH terms
Substances
Grants and funding
- T32 MH020068/MH/NIMH NIH HHS/United States
- DP2 GM105448/GM/NIGMS NIH HHS/United States
- P30 GM122732/GM/NIGMS NIH HHS/United States
- P30 GM103410/GM/NIGMS NIH HHS/United States
- P20 GM104937/GM/NIGMS NIH HHS/United States
- R01 GM118530/GM/NIGMS NIH HHS/United States
- P30 RR031153/RR/NCRR NIH HHS/United States
- R01 GM112846/GM/NIGMS NIH HHS/United States
- R35 GM119790/GM/NIGMS NIH HHS/United States
- P20 RR018728/RR/NCRR NIH HHS/United States
- R35 GM118082/GM/NIGMS NIH HHS/United States
- T32 GM007601/GM/NIGMS NIH HHS/United States
LinkOut - more resources
Full Text Sources
Other Literature Sources
Molecular Biology Databases
Research Materials
Miscellaneous