Polymeric microneedles for transdermal protein delivery
- PMID: 29408182
- PMCID: PMC6020694
- DOI: 10.1016/j.addr.2018.01.015
Polymeric microneedles for transdermal protein delivery
Abstract
The intrinsic properties of therapeutic proteins generally present a major impediment for transdermal delivery, including their relatively large molecule size and susceptibility to degradation. One solution is to utilize microneedles (MNs), which are capable of painlessly traversing the stratum corneum and directly translocating protein drugs into the systematic circulation. MNs can be designed to incorporate appropriate structural materials as well as therapeutics or formulations with tailored physicochemical properties. This platform technique has been applied to deliver drugs both locally and systemically in applications ranging from vaccination to diabetes and cancer therapy. This review surveys the current design and use of polymeric MNs for transdermal protein delivery. The clinical potential and future translation of MNs are also discussed.
Keywords: Drug delivery; Microneedle; Protein delivery; Transdermal; Vaccine.
Copyright © 2018 Elsevier B.V. All rights reserved.
Figures
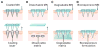
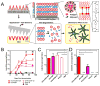
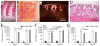
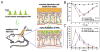
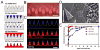
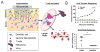
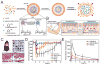
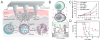
Similar articles
-
Current trends in polymer microneedle for transdermal drug delivery.Int J Pharm. 2020 Sep 25;587:119673. doi: 10.1016/j.ijpharm.2020.119673. Epub 2020 Jul 30. Int J Pharm. 2020. PMID: 32739388 Free PMC article. Review.
-
Polymeric microneedles for controlled transdermal drug delivery.J Control Release. 2019 Dec 10;315:97-113. doi: 10.1016/j.jconrel.2019.10.022. Epub 2019 Oct 20. J Control Release. 2019. PMID: 31644938 Review.
-
Microneedle Mediated Transdermal Delivery of Protein, Peptide and Antibody Based Therapeutics: Current Status and Future Considerations.Pharm Res. 2020 Jun 2;37(6):117. doi: 10.1007/s11095-020-02844-6. Pharm Res. 2020. PMID: 32488611 Free PMC article. Review.
-
Recent progress of polymeric microneedle-assisted long-acting transdermal drug delivery.J Pharm Pharm Sci. 2024 Mar 20;27:12434. doi: 10.3389/jpps.2024.12434. eCollection 2024. J Pharm Pharm Sci. 2024. PMID: 38571937 Free PMC article. Review.
-
Nanoformulation-assisted microneedle transdermal drug delivery system: An innovative platform enhancing rheumatoid arthritis treatment.Biomed Pharmacother. 2024 Sep;178:117219. doi: 10.1016/j.biopha.2024.117219. Epub 2024 Jul 30. Biomed Pharmacother. 2024. PMID: 39084080 Review.
Cited by
-
Local and Targeted Delivery of Immune Checkpoint Blockade Therapeutics.Acc Chem Res. 2020 Nov 17;53(11):2521-2533. doi: 10.1021/acs.accounts.0c00339. Epub 2020 Oct 19. Acc Chem Res. 2020. PMID: 33073988 Free PMC article.
-
Cryomicroneedles for transdermal cell delivery.Nat Biomed Eng. 2021 Sep;5(9):1008-1018. doi: 10.1038/s41551-021-00720-1. Epub 2021 May 3. Nat Biomed Eng. 2021. PMID: 33941895
-
Microneedle for transdermal drug delivery: current trends and fabrication.J Pharm Investig. 2021;51(5):503-517. doi: 10.1007/s40005-021-00512-4. Epub 2021 Mar 4. J Pharm Investig. 2021. PMID: 33686358 Free PMC article. Review.
-
Microneedle-mediated therapy for cardiovascular diseases.Drug Deliv Transl Res. 2022 Feb;12(2):472-483. doi: 10.1007/s13346-021-01073-7. Epub 2021 Oct 12. Drug Deliv Transl Res. 2022. PMID: 34637115
-
Recent Advances in Microneedle-Based Sensors for Sampling, Diagnosis and Monitoring of Chronic Diseases.Biosensors (Basel). 2021 Aug 25;11(9):296. doi: 10.3390/bios11090296. Biosensors (Basel). 2021. PMID: 34562886 Free PMC article. Review.
References
-
- Barry BW. Breaching the skin’s barrier to drugs. Nat Biotechnol. 2004;22:165–167. - PubMed
-
- Eisenstein M. Something new under the skin. Nat Biotechnol. 2011;29:107–109. - PubMed
-
- Chandrasekhar S, Iyer LK, Panchal JP, Topp EM, Cannon JB, Ranade VV. Micro-arrays and microneedle arrays for delivery of peptides, proteins, vaccines and other applications. Expert Opin Drug Deliv. 2013;10:1155–1170. - PubMed
-
- Prausnitz MR. Microneedles for transdermal drug delivery. Adv Drug Deliv Rev. 2004;56:581–587. - PubMed
-
- Park J-H, Allen MG, Prausnitz MR. Polymer microneedles for controlled-release drug delivery. Pharm Res. 2006;23:1008–1019. - PubMed
Publication types
MeSH terms
Substances
Grants and funding
LinkOut - more resources
Full Text Sources
Other Literature Sources