Molecular mechanism for the subversion of the retromer coat by the Legionella effector RidL
- PMID: 29229824
- PMCID: PMC5748213
- DOI: 10.1073/pnas.1715361115
Molecular mechanism for the subversion of the retromer coat by the Legionella effector RidL
Abstract
Microbial pathogens employ sophisticated virulence strategies to cause infections in humans. The intracellular pathogen Legionella pneumophila encodes RidL to hijack the host scaffold protein VPS29, a component of retromer and retriever complexes critical for endosomal cargo recycling. Here, we determined the crystal structure of L. pneumophila RidL in complex with the human VPS29-VPS35 retromer subcomplex. A hairpin loop protruding from RidL inserts into a conserved pocket on VPS29 that is also used by cellular ligands, such as Tre-2/Bub2/Cdc16 domain family member 5 (TBC1D5) and VPS9-ankyrin repeat protein for VPS29 binding. Consistent with the idea of molecular mimicry in protein interactions, RidL outcompeted TBC1D5 for binding to VPS29. Furthermore, the interaction of RidL with retromer did not interfere with retromer dimerization but was essential for association of RidL with retromer-coated vacuolar and tubular endosomes. Our work thus provides structural and mechanistic evidence into how RidL is targeted to endosomal membranes.
Keywords: Legionella effector; X-ray crystallography; coat complex; membrane targeting; pathogenic bacteria.
Copyright © 2017 the Author(s). Published by PNAS.
Conflict of interest statement
The authors declare no conflict of interest.
Figures
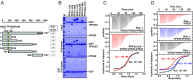
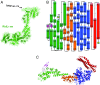
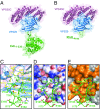
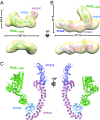
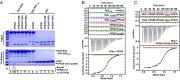
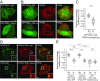
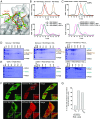
Similar articles
-
Mechanism of inhibition of retromer transport by the bacterial effector RidL.Proc Natl Acad Sci U S A. 2018 Feb 13;115(7):E1446-E1454. doi: 10.1073/pnas.1717383115. Epub 2018 Jan 31. Proc Natl Acad Sci U S A. 2018. PMID: 29386389 Free PMC article.
-
Structural insights into Legionella RidL-Vps29 retromer subunit interaction reveal displacement of the regulator TBC1D5.Nat Commun. 2017 Nov 16;8(1):1543. doi: 10.1038/s41467-017-01512-5. Nat Commun. 2017. PMID: 29146912 Free PMC article.
-
The Legionella effector RidL inhibits retrograde trafficking to promote intracellular replication.Cell Host Microbe. 2013 Jul 17;14(1):38-50. doi: 10.1016/j.chom.2013.06.001. Cell Host Microbe. 2013. PMID: 23870312
-
Emerging Role of Retromer in Modulating Pathogen Growth.Trends Microbiol. 2018 Sep;26(9):769-780. doi: 10.1016/j.tim.2018.04.001. Epub 2018 Apr 24. Trends Microbiol. 2018. PMID: 29703496 Free PMC article. Review.
-
Formation of the Legionella Replicative Compartment at the Crossroads of Retrograde Trafficking.Front Cell Infect Microbiol. 2017 Nov 24;7:482. doi: 10.3389/fcimb.2017.00482. eCollection 2017. Front Cell Infect Microbiol. 2017. PMID: 29226112 Free PMC article. Review.
Cited by
-
A Role for the VPS Retromer in Brucella Intracellular Replication Revealed by Genomewide siRNA Screening.mSphere. 2019 Jun 26;4(3):e00380-19. doi: 10.1128/mSphere.00380-19. mSphere. 2019. PMID: 31243080 Free PMC article.
-
Study of Legionella Effector Domains Revealed Novel and Prevalent Phosphatidylinositol 3-Phosphate Binding Domains.Infect Immun. 2019 May 21;87(6):e00153-19. doi: 10.1128/IAI.00153-19. Print 2019 Jun. Infect Immun. 2019. PMID: 30962397 Free PMC article.
-
Eat or Be Eaten: Strategies Used by Legionella to Acquire Host-Derived Nutrients and Evade Lysosomal Degradation.Infect Immun. 2023 Apr 18;91(4):e0044122. doi: 10.1128/iai.00441-22. Epub 2023 Mar 13. Infect Immun. 2023. PMID: 36912646 Free PMC article. Review.
-
Structure of the membrane-assembled retromer coat determined by cryo-electron tomography.Nature. 2018 Sep;561(7724):561-564. doi: 10.1038/s41586-018-0526-z. Epub 2018 Sep 17. Nature. 2018. PMID: 30224749 Free PMC article.
-
Pathogenicity and Virulence of Legionella: Intracellular replication and host response.Virulence. 2021 Dec;12(1):1122-1144. doi: 10.1080/21505594.2021.1903199. Virulence. 2021. PMID: 33843434 Free PMC article. Review.
References
-
- Scott CC, Vacca F, Gruenberg J. Endosome maturation, transport and functions. Semin Cell Dev Biol. 2014;31:2–10. - PubMed
-
- Hierro A, Gershlick DC, Rojas AL, Bonifacino JS. Formation of tubulovesicular carriers from endosomes and their fusion to the trans-Golgi network. Int Rev Cell Mol Biol. 2015;318:159–202. - PubMed
Publication types
MeSH terms
Substances
Associated data
- Actions
- Actions
- Actions
Grants and funding
LinkOut - more resources
Full Text Sources
Other Literature Sources
Research Materials
Miscellaneous