Metabolic activity induces membrane phase separation in endoplasmic reticulum
- PMID: 29196526
- PMCID: PMC5754785
- DOI: 10.1073/pnas.1712555114
Metabolic activity induces membrane phase separation in endoplasmic reticulum
Abstract
Membrane phase behavior has been well characterized in model membranes in vitro under thermodynamic equilibrium state. However, the widely observed differences between biological membranes and their in vitro counterparts are placing more emphasis on nonequilibrium factors, including influx and efflux of lipid molecules. The endoplasmic reticulum (ER) is the largest cellular membrane system and also the most metabolically active organelle responsible for lipid synthesis. However, how the nonequilibrium metabolic activity modulates ER membrane phase has not been investigated. Here, we studied the phase behavior of functional ER in the context of lipid metabolism. Utilizing advanced vibrational imaging technique, that is, stimulated Raman scattering microscopy, we discovered that metabolism of palmitate, a prevalent saturated fatty acid (SFA), could drive solid-like domain separation from the presumably uniformly fluidic ER membrane, a previously unknown phenomenon. The potential of various fatty acids to induce solid phase can be predicted by the transition temperatures of their major metabolites. Interplay between saturated and unsaturated fatty acids is also observed. Hence, our study sheds light on cellular membrane biophysics by underscoring the nonequilibrium metabolic status of living cell.
Keywords: Raman imaging; endoplasmic reticulum; fatty acid; lipid metabolism; membrane phase.
Copyright © 2017 the Author(s). Published by PNAS.
Conflict of interest statement
The authors declare no conflict of interest.
Figures
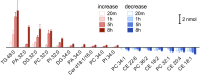
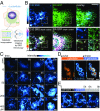
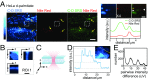
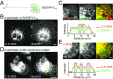
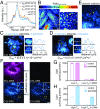
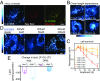
Similar articles
-
VMP1 Establishes ER-Microdomains that Regulate Membrane Contact Sites and Autophagy.PLoS One. 2016 Nov 18;11(11):e0166499. doi: 10.1371/journal.pone.0166499. eCollection 2016. PLoS One. 2016. PMID: 27861594 Free PMC article.
-
Saturated fatty acids induce endoplasmic reticulum stress and apoptosis independently of ceramide in liver cells.Am J Physiol Endocrinol Metab. 2006 Aug;291(2):E275-81. doi: 10.1152/ajpendo.00644.2005. Epub 2006 Feb 21. Am J Physiol Endocrinol Metab. 2006. PMID: 16492686
-
Three-dimensional architecture of extended synaptotagmin-mediated endoplasmic reticulum-plasma membrane contact sites.Proc Natl Acad Sci U S A. 2015 Apr 21;112(16):E2004-13. doi: 10.1073/pnas.1503191112. Epub 2015 Mar 18. Proc Natl Acad Sci U S A. 2015. PMID: 25787254 Free PMC article.
-
Endoplasmic Reticulum-Mitochondria Communication Through Ca2+ Signaling: The Importance of Mitochondria-Associated Membranes (MAMs).Adv Exp Med Biol. 2017;997:49-67. doi: 10.1007/978-981-10-4567-7_4. Adv Exp Med Biol. 2017. PMID: 28815521 Review.
-
Endoplasmic Reticulum - Plasma Membrane Crosstalk Mediated by the Extended Synaptotagmins.Adv Exp Med Biol. 2017;997:83-93. doi: 10.1007/978-981-10-4567-7_6. Adv Exp Med Biol. 2017. PMID: 28815523 Review.
Cited by
-
A primary effect of palmitic acid on mouse oocytes is the disruption of the structure of the endoplasmic reticulum.Reproduction. 2021 Dec 28;163(1):45-56. doi: 10.1530/REP-21-0332. Reproduction. 2021. PMID: 34866595 Free PMC article.
-
Alleviation of Postharvest Chilling Injury of Carambola Fruit by γ-aminobutyric Acid: Physiological, Biochemical, and Structural Characterization.Front Nutr. 2021 Nov 16;8:752583. doi: 10.3389/fnut.2021.752583. eCollection 2021. Front Nutr. 2021. PMID: 34869526 Free PMC article.
-
Bringing light to ER contacts and a new phase in organelle communication.Proc Natl Acad Sci U S A. 2020 May 5;117(18):9668-9670. doi: 10.1073/pnas.2003620117. Epub 2020 Apr 28. Proc Natl Acad Sci U S A. 2020. PMID: 32345722 Free PMC article. No abstract available.
-
Greasing the Wheels of Lipid Biology with Chemical Tools.Trends Biochem Sci. 2018 Dec;43(12):970-983. doi: 10.1016/j.tibs.2018.09.011. Epub 2018 Oct 26. Trends Biochem Sci. 2018. PMID: 30472989 Free PMC article. Review.
-
Visualizing Subcellular Enrichment of Glycogen in Live Cancer Cells by Stimulated Raman Scattering.Anal Chem. 2020 Oct 6;92(19):13182-13191. doi: 10.1021/acs.analchem.0c02348. Epub 2020 Sep 21. Anal Chem. 2020. PMID: 32907318 Free PMC article.
References
Publication types
MeSH terms
Substances
Grants and funding
LinkOut - more resources
Full Text Sources
Other Literature Sources