Crystallizable Fragment Glycoengineering for Therapeutic Antibodies Development
- PMID: 29181010
- PMCID: PMC5693878
- DOI: 10.3389/fimmu.2017.01554
Crystallizable Fragment Glycoengineering for Therapeutic Antibodies Development
Abstract
Monoclonal antibody (mAb)-based therapeutics are the fastest growing class of human pharmaceuticals. They are typically IgG1 molecules with N-glycans attached to the N297 residue on crystallizable fragment (Fc). Different Fc glycoforms impact their effector function, pharmacokinetics, stability, aggregation, safety, and immunogenicity. Fc glycoforms affect mAbs effector functions including antibody-dependent cell-mediated cytotoxicity (ADCC) and complement-dependent cytotoxicity (CDC) by modulating the Fc-FcγRs and Fc-C1q interactions. While the terminal galactose enhances CDC activity, the fucose significantly decreases ADCC. Defucosylated immunoglobulin Gs (IgGs) are thus highly pursued as next-generation therapeutic mAbs with potent ADCC at reduced doses. A plethora of cell glycoengineering and chemoenzymatic glycoengineering strategies is emerging to produce IgGs with homogenous glycoforms especially without core fucose. The chemoenzymatic glycosylation remodeling also offers useful avenues for site-specific conjugations of small molecule drugs onto mAbs. Herein, we review the current progress of IgG-Fc glycoengineering. We begin with the discussion of the structures of IgG N-glycans and biosynthesis followed by reviewing the impact of IgG glycoforms on antibody effector functions and the current Fc glycoengineering strategies with emphasis on Fc defucosylation. Furthermore, we briefly discuss two novel therapeutic mAbs formats: aglycosylated mAbs and Fc glycan specific antibody-drug conjugates (ADCs). The advances in the understanding of Fc glycobiology and development of novel glycoengineering technologies have facilitated the generation of therapeutic mAbs with homogenous glycoforms and improved therapeutic efficacy.
Keywords: aglycosylated monoclonal antibodies; antibody–drug conjugate; chemoenzymatic glycosylation remodeling; crystallizable fragment glycoengineering; crystallizable fragment glycosylation; effector function; homogenous glycoforms; monoclonal antibodies.
Figures
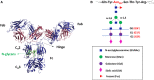
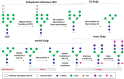
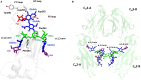
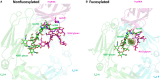
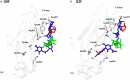
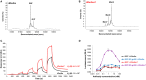
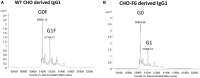
Similar articles
-
Effects of terminal galactose residues in mannose α1-6 arm of Fc-glycan on the effector functions of therapeutic monoclonal antibodies.MAbs. 2019 Jul;11(5):826-836. doi: 10.1080/19420862.2019.1608143. Epub 2019 May 8. MAbs. 2019. PMID: 30990348 Free PMC article.
-
Influence of N-glycosylation on effector functions and thermal stability of glycoengineered IgG1 monoclonal antibody with homogeneous glycoforms.MAbs. 2019 Feb/Mar;11(2):350-372. doi: 10.1080/19420862.2018.1551044. Epub 2018 Dec 10. MAbs. 2019. PMID: 30466347 Free PMC article.
-
Principles of N-Linked Glycosylation Variations of IgG-Based Therapeutics: Pharmacokinetic and Functional Considerations.Antibodies (Basel). 2020 Jun 10;9(2):22. doi: 10.3390/antib9020022. Antibodies (Basel). 2020. PMID: 32532067 Free PMC article. Review.
-
Chemoenzymatic glycoengineering of intact IgG antibodies for gain of functions.J Am Chem Soc. 2012 Jul 25;134(29):12308-18. doi: 10.1021/ja3051266. Epub 2012 Jul 16. J Am Chem Soc. 2012. PMID: 22747414 Free PMC article.
-
Importance and Monitoring of Therapeutic Immunoglobulin G Glycosylation.Exp Suppl. 2021;112:481-517. doi: 10.1007/978-3-030-76912-3_15. Exp Suppl. 2021. PMID: 34687020 Review.
Cited by
-
Establishment of a highly precise multi-attribute method for the characterization and quality control of therapeutic monoclonal antibodies.Bioengineered. 2020 Dec;11(1):984-1000. doi: 10.1080/21655979.2020.1814683. Bioengineered. 2020. PMID: 32942957 Free PMC article.
-
Simplifying the detection and monitoring of protein glycosylation during in vitro glycoengineering.Sci Rep. 2023 Jan 11;13(1):567. doi: 10.1038/s41598-023-27634-z. Sci Rep. 2023. PMID: 36631484 Free PMC article.
-
Glycans in drug discovery.Medchemcomm. 2019 Jul 26;10(10):1678-1691. doi: 10.1039/c9md00292h. eCollection 2019 Oct 1. Medchemcomm. 2019. PMID: 31814952 Free PMC article. Review.
-
The Contribution of IgG Glycosylation to Antibody-Dependent Cell-Mediated Cytotoxicity (ADCC) and Complement-Dependent Cytotoxicity (CDC) in Hashimoto's Thyroiditis: An in Vitro Model of Thyroid Autoimmunity.Biomolecules. 2020 Jan 22;10(2):171. doi: 10.3390/biom10020171. Biomolecules. 2020. PMID: 31979029 Free PMC article.
-
Improving Immunotherapy Through Glycodesign.Front Immunol. 2018 Nov 2;9:2485. doi: 10.3389/fimmu.2018.02485. eCollection 2018. Front Immunol. 2018. PMID: 30450094 Free PMC article. Review.
References
Publication types
LinkOut - more resources
Full Text Sources
Other Literature Sources