Partitioning of One-Carbon Units in Folate and Methionine Metabolism Is Essential for Neural Tube Closure
- PMID: 29141214
- PMCID: PMC5699646
- DOI: 10.1016/j.celrep.2017.10.072
Partitioning of One-Carbon Units in Folate and Methionine Metabolism Is Essential for Neural Tube Closure
Abstract
Abnormal folate one-carbon metabolism (FOCM) is implicated in neural tube defects (NTDs), severe malformations of the nervous system. MTHFR mediates unidirectional transfer of methyl groups from the folate cycle to the methionine cycle and, therefore, represents a key nexus in partitioning one-carbon units between FOCM functional outputs. Methionine cycle inhibitors prevent neural tube closure in mouse embryos. Similarly, the inability to use glycine as a one-carbon donor to the folate cycle causes NTDs in glycine decarboxylase (Gldc)-deficient embryos. However, analysis of Mthfr-null mouse embryos shows that neither S-adenosylmethionine abundance nor neural tube closure depend on one-carbon units derived from embryonic or maternal folate cycles. Mthfr deletion or methionine treatment prevents NTDs in Gldc-null embryos by retention of one-carbon units within the folate cycle. Overall, neural tube closure depends on the activity of both the methionine and folate cycles, but transfer of one-carbon units between the cycles is not necessary.
Keywords: Gldc; Mthfr; eye; folic acid; glycine cleavage system; neural tube defects; non-ketotic hyperglycinemia; one-carbon metabolism; spina bifida.
Copyright © 2017 The Author(s). Published by Elsevier Inc. All rights reserved.
Figures
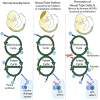
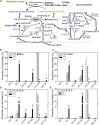
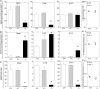
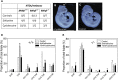

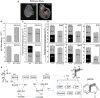
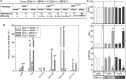
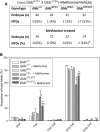
Similar articles
-
Neural tube defects induced by folate deficiency in mutant curly tail (Grhl3) embryos are associated with alteration in folate one-carbon metabolism but are unlikely to result from diminished methylation.Birth Defects Res A Clin Mol Teratol. 2010 Aug;88(8):612-8. doi: 10.1002/bdra.20690. Birth Defects Res A Clin Mol Teratol. 2010. PMID: 20589880 Free PMC article.
-
Impaired folate 1-carbon metabolism causes formate-preventable hydrocephalus in glycine decarboxylase-deficient mice.J Clin Invest. 2020 Mar 2;130(3):1446-1452. doi: 10.1172/JCI132360. J Clin Invest. 2020. PMID: 31794432 Free PMC article.
-
Glycine decarboxylase deficiency causes neural tube defects and features of non-ketotic hyperglycinemia in mice.Nat Commun. 2015 Mar 4;6:6388. doi: 10.1038/ncomms7388. Nat Commun. 2015. PMID: 25736695 Free PMC article.
-
Insights into prevention of human neural tube defects by folic acid arising from consideration of mouse mutants.Birth Defects Res A Clin Mol Teratol. 2009 Apr;85(4):331-9. doi: 10.1002/bdra.20552. Birth Defects Res A Clin Mol Teratol. 2009. PMID: 19117321 Review.
-
Folate receptors and neural tube closure.Congenit Anom (Kyoto). 2017 Sep;57(5):130-133. doi: 10.1111/cga.12218. Epub 2017 Apr 18. Congenit Anom (Kyoto). 2017. PMID: 28244241 Review.
Cited by
-
Regulation of glycine metabolism by the glycine cleavage system and conjugation pathway in mouse models of non-ketotic hyperglycinemia.J Inherit Metab Dis. 2020 Nov;43(6):1186-1198. doi: 10.1002/jimd.12295. Epub 2020 Aug 11. J Inherit Metab Dis. 2020. PMID: 32743799 Free PMC article.
-
Abnormal methylation caused by folic acid deficiency in neural tube defects.Open Life Sci. 2022 Dec 22;17(1):1679-1688. doi: 10.1515/biol-2022-0504. eCollection 2022. Open Life Sci. 2022. PMID: 36589786 Free PMC article. Review.
-
Modeling Rare Human Disorders in Mice: The Finnish Disease Heritage.Cells. 2021 Nov 13;10(11):3158. doi: 10.3390/cells10113158. Cells. 2021. PMID: 34831381 Free PMC article. Review.
-
Measurement of Melanin Metabolism in Live Cells by [U-13C]-L-Tyrosine Fate Tracing Using Liquid Chromatography-Mass Spectrometry.J Invest Dermatol. 2021 Jul;141(7):1810-1818.e6. doi: 10.1016/j.jid.2021.01.007. Epub 2021 Feb 5. J Invest Dermatol. 2021. PMID: 33549605 Free PMC article.
-
Ketogenic diet as a glycine lowering therapy in nonketotic hyperglycinemia and impact on brain glycine levels.Orphanet J Rare Dis. 2022 Dec 5;17(1):423. doi: 10.1186/s13023-022-02581-6. Orphanet J Rare Dis. 2022. PMID: 36471344 Free PMC article.
References
-
- Afman L.A., Blom H.J., Drittij M.J., Brouns M.R., van Straaten H.W. Inhibition of transmethylation disturbs neurulation in chick embryos. Brain Res. Dev. Brain Res. 2005;158:59–65. - PubMed
-
- Botto L.D., Yang Q. 5,10-Methylenetetrahydrofolate reductase gene variants and congenital anomalies: a HuGE review. Am. J. Epidemiol. 2000;151:862–877. - PubMed
MeSH terms
Substances
Grants and funding
- NC/M001555/1/NC3RS_/National Centre for the Replacement, Refinement and Reduction of Animals in Research/United Kingdom
- MR/N003713/1/MRC_/Medical Research Council/United Kingdom
- J003794/MRC_/Medical Research Council/United Kingdom
- G0401315/MRC_/Medical Research Council/United Kingdom
- P01 HD067244/HD/NICHD NIH HHS/United States
LinkOut - more resources
Full Text Sources
Other Literature Sources
Medical
Molecular Biology Databases