Dynamic changes in eIF4F-mRNA interactions revealed by global analyses of environmental stress responses
- PMID: 29078784
- PMCID: PMC5660459
- DOI: 10.1186/s13059-017-1338-4
Dynamic changes in eIF4F-mRNA interactions revealed by global analyses of environmental stress responses
Abstract
Background: Translation factors eIF4E and eIF4G form eIF4F, which interacts with the messenger RNA (mRNA) 5' cap to promote ribosome recruitment and translation initiation. Variations in the association of eIF4F with individual mRNAs likely contribute to differences in translation initiation frequencies between mRNAs. As translation initiation is globally reprogrammed by environmental stresses, we were interested in determining whether eIF4F interactions with individual mRNAs are reprogrammed and how this may contribute to global environmental stress responses.
Results: Using a tagged-factor protein capture and RNA-sequencing (RNA-seq) approach, we have assessed how mRNA associations with eIF4E, eIF4G1 and eIF4G2 change globally in response to three defined stresses that each cause a rapid attenuation of protein synthesis: oxidative stress induced by hydrogen peroxide and nutrient stresses caused by amino acid or glucose withdrawal. We find that acute stress leads to dynamic and unexpected changes in eIF4F-mRNA interactions that are shared among each factor and across the stresses imposed. eIF4F-mRNA interactions stabilised by stress are predominantly associated with translational repression, while more actively initiating mRNAs become relatively depleted for eIF4F. Simultaneously, other mRNAs are insulated from these stress-induced changes in eIF4F association.
Conclusion: Dynamic eIF4F-mRNA interaction changes are part of a coordinated early translational control response shared across environmental stresses. Our data are compatible with a model where multiple mRNA closed-loop complexes form with differing stability. Hence, unexpectedly, in the absence of other stabilising factors, rapid translation initiation on mRNAs correlates with less stable eIF4F interactions.
Keywords: Stress regulation of gene expression; Translational control; Yeast; eIF4F.
Conflict of interest statement
Ethics approval and consent to participate
This study did not require ethical approval.
Competing interests
The authors declare that they have no competing interests.
Publisher’s Note
Springer Nature remains neutral with regard to jurisdictional claims in published maps and institutional affiliations.
Figures
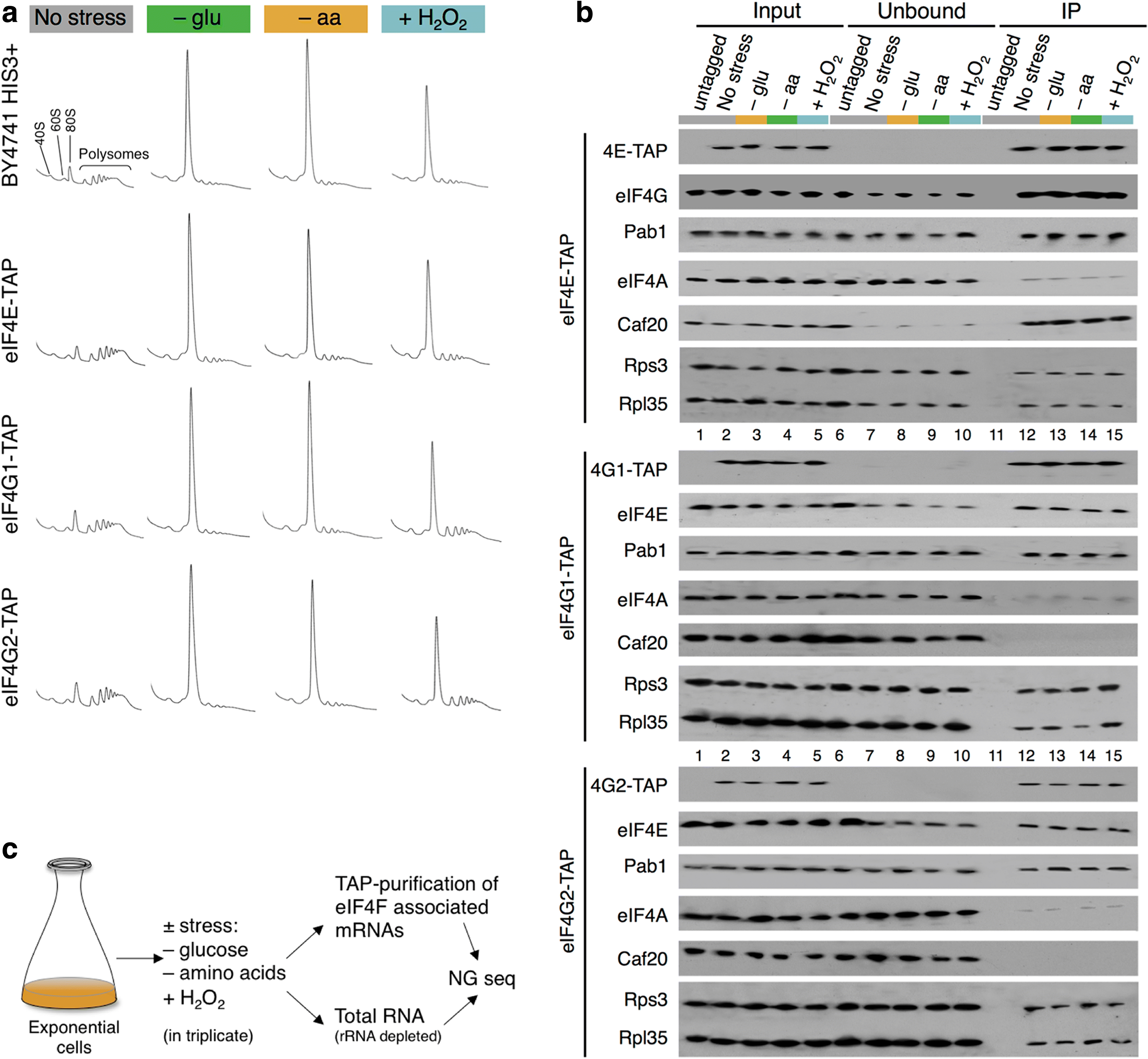


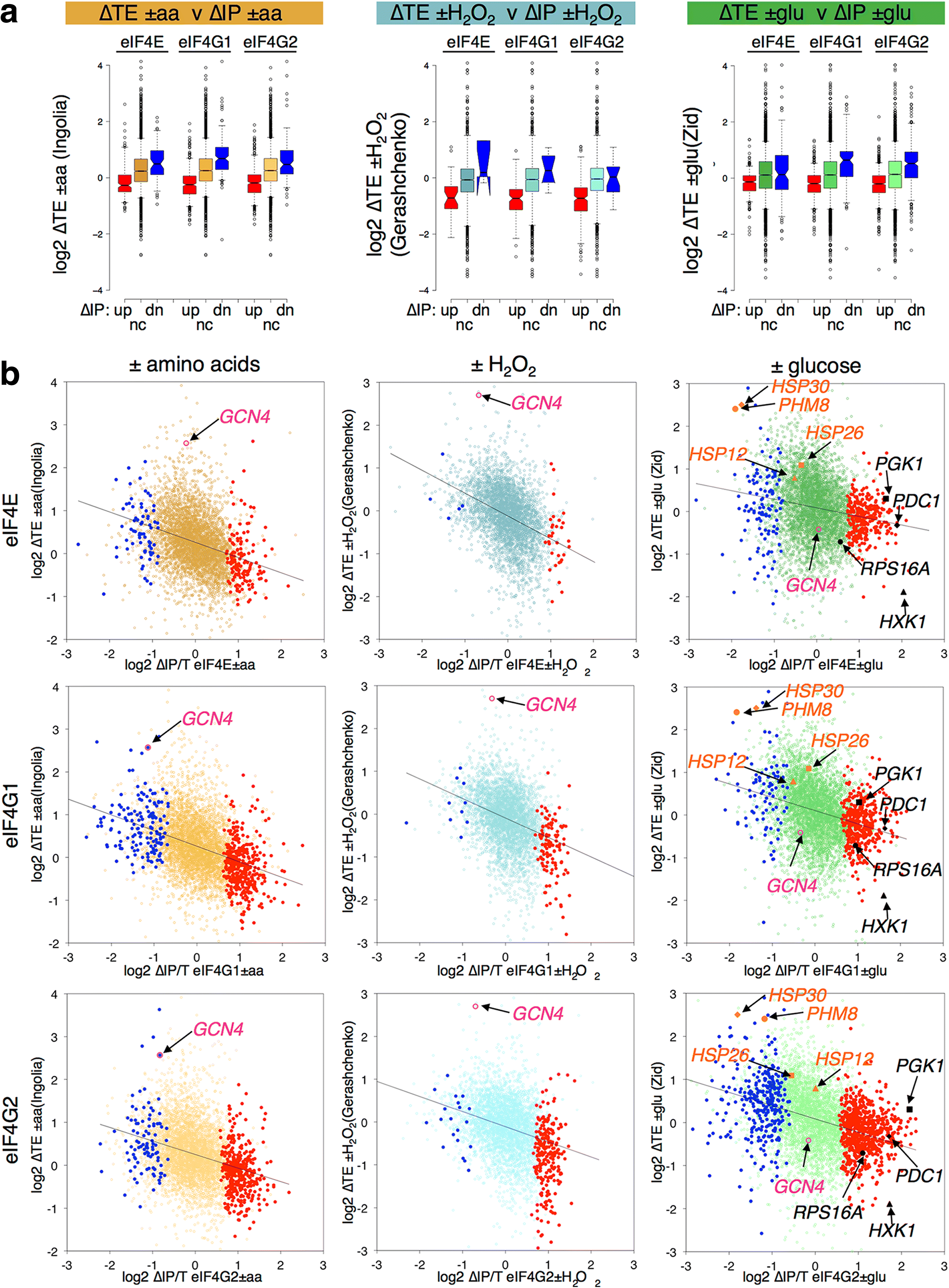
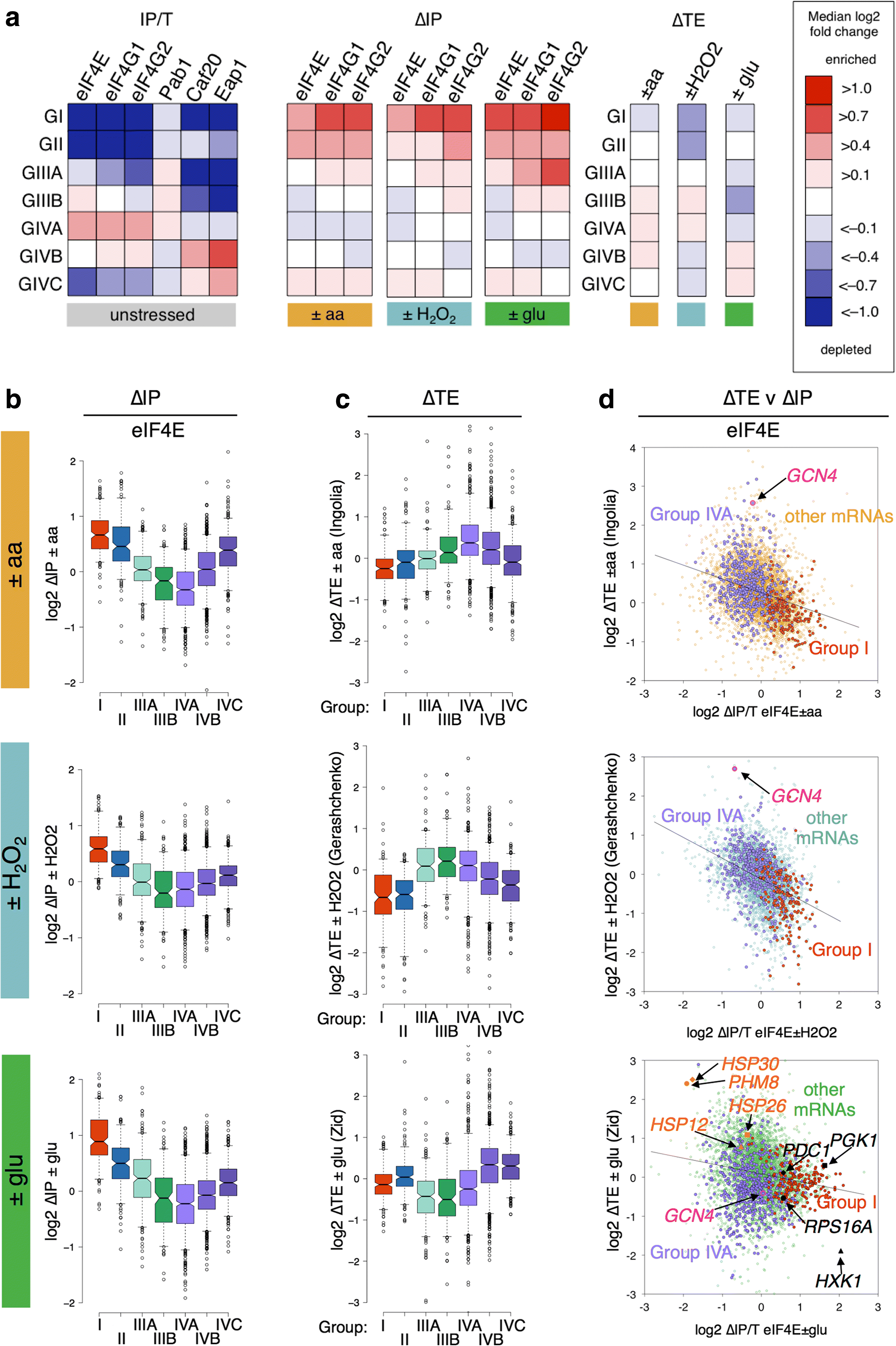
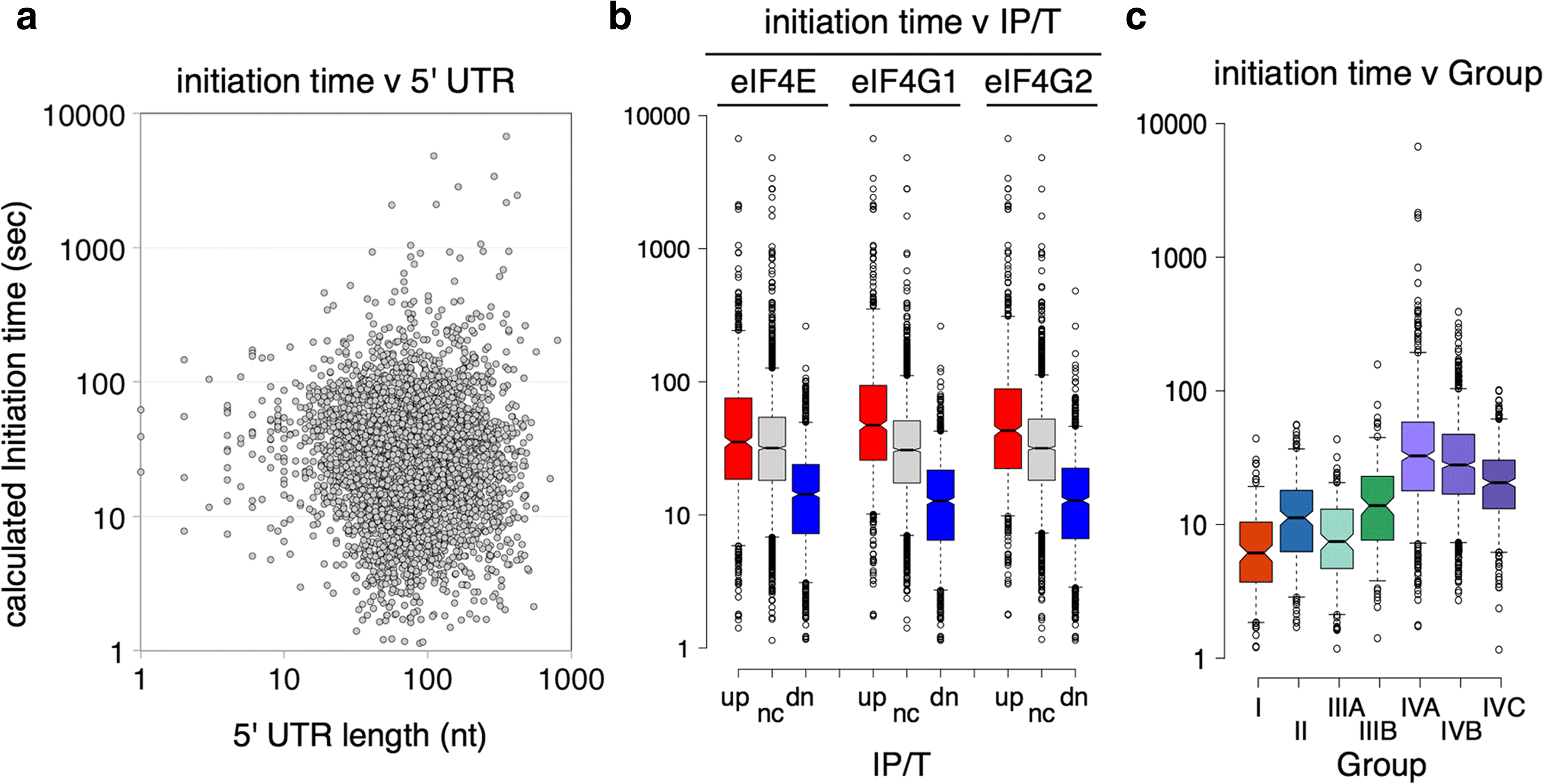

Similar articles
-
mRNA- and factor-driven dynamic variability controls eIF4F-cap recognition for translation initiation.Nucleic Acids Res. 2022 Aug 12;50(14):8240-8261. doi: 10.1093/nar/gkac631. Nucleic Acids Res. 2022. PMID: 35871304 Free PMC article.
-
Specific domains in yeast translation initiation factor eIF4G strongly bias RNA unwinding activity of the eIF4F complex toward duplexes with 5'-overhangs.J Biol Chem. 2012 Jun 8;287(24):20301-12. doi: 10.1074/jbc.M112.347278. Epub 2012 Mar 30. J Biol Chem. 2012. PMID: 22467875 Free PMC article.
-
Toward the mechanism of eIF4F-mediated ribosomal attachment to mammalian capped mRNAs.Genes Dev. 2016 Jul 1;30(13):1573-88. doi: 10.1101/gad.282418.116. Genes Dev. 2016. PMID: 27401559 Free PMC article.
-
eIF4 initiation factors: effectors of mRNA recruitment to ribosomes and regulators of translation.Annu Rev Biochem. 1999;68:913-63. doi: 10.1146/annurev.biochem.68.1.913. Annu Rev Biochem. 1999. PMID: 10872469 Review.
-
Regulation of translation initiation by amino acids in eukaryotic cells.Prog Mol Subcell Biol. 2001;26:155-84. doi: 10.1007/978-3-642-56688-2_6. Prog Mol Subcell Biol. 2001. PMID: 11575165 Review.
Cited by
-
Yeast Secretes High Amounts of Human Calreticulin without Cellular Stress.Curr Issues Mol Biol. 2022 Apr 19;44(5):1768-1787. doi: 10.3390/cimb44050122. Curr Issues Mol Biol. 2022. PMID: 35678651 Free PMC article.
-
Functional interplay between DEAD-box RNA helicases Ded1 and Dbp1 in preinitiation complex attachment and scanning on structured mRNAs in vivo.Nucleic Acids Res. 2019 Sep 19;47(16):8785-8806. doi: 10.1093/nar/gkz595. Nucleic Acids Res. 2019. PMID: 31299079 Free PMC article.
-
Protein Synthesis Initiation in Eukaryotic Cells.Cold Spring Harb Perspect Biol. 2018 Dec 3;10(12):a033092. doi: 10.1101/cshperspect.a033092. Cold Spring Harb Perspect Biol. 2018. PMID: 29735639 Free PMC article. Review.
-
RNAs: dynamic and mutable.Genome Biol. 2017 Nov 29;18(1):226. doi: 10.1186/s13059-017-1361-5. Genome Biol. 2017. PMID: 29187228 Free PMC article. No abstract available.
-
Archetypal transcriptional blocks underpin yeast gene regulation in response to changes in growth conditions.Sci Rep. 2018 May 21;8(1):7949. doi: 10.1038/s41598-018-26170-5. Sci Rep. 2018. PMID: 29785040 Free PMC article.
References
MeSH terms
Substances
Grants and funding
LinkOut - more resources
Full Text Sources
Other Literature Sources
Molecular Biology Databases
Research Materials
Miscellaneous