Direction of leukocyte polarization and migration by the phosphoinositide-transfer protein TIPE2
- PMID: 29058702
- PMCID: PMC5690821
- DOI: 10.1038/ni.3866
Direction of leukocyte polarization and migration by the phosphoinositide-transfer protein TIPE2
Abstract
The polarization of leukocytes toward chemoattractants is essential for the directed migration (chemotaxis) of leukocytes. How leukocytes acquire polarity after encountering chemical gradients is not well understood. We found here that leukocyte polarity was generated by TIPE2 (TNFAIP8L2), a transfer protein for phosphoinositide second messengers. TIPE2 functioned as a local enhancer of phosphoinositide-dependent signaling and cytoskeleton remodeling, which promoted leading-edge formation. Conversely, TIPE2 acted as an inhibitor of the GTPase Rac, which promoted trailing-edge polarization. Consequently, TIPE2-deficient leukocytes were defective in polarization and chemotaxis, and TIPE2-deficient mice were resistant to leukocyte-mediated neural inflammation. Thus, the leukocyte polarizer is a dual-role phosphoinositide-transfer protein and represents a potential therapeutic target for the treatment of inflammatory diseases.
Conflict of interest statement
The authors have no financial conflict of interest with this work.
Figures
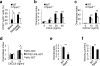
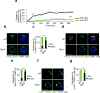
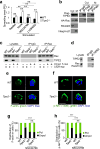
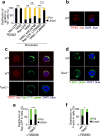
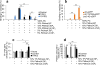
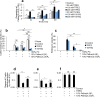
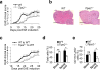
Similar articles
-
TIPE2 controls innate immunity to RNA by targeting the phosphatidylinositol 3-kinase-Rac pathway.J Immunol. 2012 Sep 15;189(6):2768-73. doi: 10.4049/jimmunol.1103477. Epub 2012 Aug 17. J Immunol. 2012. PMID: 22904303 Free PMC article.
-
TIPE2 specifies the functional polarization of myeloid-derived suppressor cells during tumorigenesis.J Exp Med. 2020 Feb 3;217(2):e20182005. doi: 10.1084/jem.20182005. J Exp Med. 2020. PMID: 31662347 Free PMC article.
-
Egress of murine regulatory T cells from the thymus requires TIPE2.Biochem Biophys Res Commun. 2018 Jun 2;500(2):376-383. doi: 10.1016/j.bbrc.2018.04.082. Epub 2018 Apr 14. Biochem Biophys Res Commun. 2018. PMID: 29654762
-
Do phosphoinositide 3-kinases direct lymphocyte navigation?Trends Immunol. 2004 Feb;25(2):67-74. doi: 10.1016/j.it.2003.12.003. Trends Immunol. 2004. PMID: 15102365 Review.
-
Lipids on the move: phosphoinositide 3-kinases in leukocyte function.Immunol Today. 2000 Jun;21(6):260-4. doi: 10.1016/s0167-5699(00)01649-2. Immunol Today. 2000. PMID: 10939787 Review. No abstract available.
Cited by
-
TIPE proteins control directed migration of human T cells by directing GPCR and lipid second messenger signaling.J Leukoc Biol. 2024 Feb 23;115(3):511-524. doi: 10.1093/jleuko/qiad141. J Leukoc Biol. 2024. PMID: 37952106 Free PMC article.
-
TNFAIP8 family gene expressions in the mouse tail intervertebral disc injury model.JOR Spine. 2020 Jun 19;3(2):e1093. doi: 10.1002/jsp2.1093. eCollection 2020 Jun. JOR Spine. 2020. PMID: 32613168 Free PMC article.
-
Autophagic lysosome reformation in health and disease.Autophagy. 2023 May;19(5):1378-1395. doi: 10.1080/15548627.2022.2128019. Epub 2022 Nov 21. Autophagy. 2023. PMID: 36409033 Free PMC article. Review.
-
TIPE2 May Target the Nrf2/HO-1 Pathway to Inhibit M1 Macrophage-Related Neutrophilic Inflammation in Asthma.Front Immunol. 2022 Apr 28;13:883885. doi: 10.3389/fimmu.2022.883885. eCollection 2022. Front Immunol. 2022. PMID: 35572500 Free PMC article.
-
TIPE2 Promotes Tumor Initiation But Inhibits Tumor Progression in Murine Colitis-Associated Colon Cancer.Inflamm Bowel Dis. 2022 May 4;28(5):764-774. doi: 10.1093/ibd/izab306. Inflamm Bowel Dis. 2022. PMID: 34894222 Free PMC article.
References
-
- Merlot S, Firtel RA. Leading the way: Directional sensing through phosphatidylinositol 3-kinase and other signaling pathways. J. Cell Sci. 2003;116:3471–3478. - PubMed
MeSH terms
Substances
Grants and funding
LinkOut - more resources
Full Text Sources
Other Literature Sources
Molecular Biology Databases
Miscellaneous