Natively Unfolded FG Repeats Stabilize the Structure of the Nuclear Pore Complex
- PMID: 29033133
- PMCID: PMC5992322
- DOI: 10.1016/j.cell.2017.09.033
Natively Unfolded FG Repeats Stabilize the Structure of the Nuclear Pore Complex
Abstract
Nuclear pore complexes (NPCs) are ∼100 MDa transport channels assembled from multiple copies of ∼30 nucleoporins (Nups). One-third of these Nups contain phenylalanine-glycine (FG)-rich repeats, forming a diffusion barrier, which is selectively permeable for nuclear transport receptors that interact with these repeats. Here, we identify an additional function of FG repeats in the structure and biogenesis of the yeast NPC. We demonstrate that GLFG-containing FG repeats directly bind to multiple scaffold Nups in vitro and act as NPC-targeting determinants in vivo. Furthermore, we show that the GLFG repeats of Nup116 function in a redundant manner with Nup188, a nonessential scaffold Nup, to stabilize critical interactions within the NPC scaffold needed for late steps of NPC assembly. Our results reveal a previously unanticipated structural role for natively unfolded GLFG repeats as Velcro to link NPC subcomplexes and thus add a new layer of connections to current models of the NPC architecture.
Keywords: FG repeats; Intrinsically disordered domains; Nuclear envelope; Nuclear pore biogenesis; Nuclear pore complex; Nuclear pore structure; Protein interactions.
Copyright © 2017 Elsevier Inc. All rights reserved.
Figures
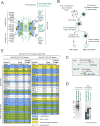
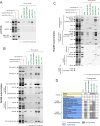
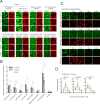
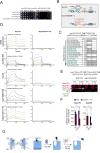
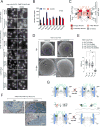
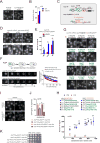
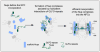
Similar articles
-
Nucleoporin's Like Charge Regions Are Major Regulators of FG Coverage and Dynamics Inside the Nuclear Pore Complex.PLoS One. 2015 Dec 11;10(12):e0143745. doi: 10.1371/journal.pone.0143745. eCollection 2015. PLoS One. 2015. PMID: 26658558 Free PMC article.
-
Natively unfolded nucleoporins gate protein diffusion across the nuclear pore complex.Cell. 2007 Apr 6;129(1):83-96. doi: 10.1016/j.cell.2007.01.044. Cell. 2007. PMID: 17418788
-
Entry into the nuclear pore complex is controlled by a cytoplasmic exclusion zone containing dynamic GLFG-repeat nucleoporin domains.J Cell Sci. 2014 Jan 1;127(Pt 1):124-36. doi: 10.1242/jcs.133272. Epub 2013 Oct 21. J Cell Sci. 2014. PMID: 24144701
-
Biomechanics of the transport barrier in the nuclear pore complex.Semin Cell Dev Biol. 2017 Aug;68:42-51. doi: 10.1016/j.semcdb.2017.05.007. Epub 2017 May 12. Semin Cell Dev Biol. 2017. PMID: 28506890 Review.
-
The selective permeability barrier in the nuclear pore complex.Nucleus. 2016 Sep 2;7(5):430-446. doi: 10.1080/19491034.2016.1238997. Epub 2016 Sep 27. Nucleus. 2016. PMID: 27673359 Free PMC article. Review.
Cited by
-
Nuclear Pores Assemble from Nucleoporin Condensates During Oogenesis.Cell. 2019 Oct 17;179(3):671-686.e17. doi: 10.1016/j.cell.2019.09.022. Cell. 2019. PMID: 31626769 Free PMC article.
-
C9orf72 arginine-rich dipeptide repeat proteins disrupt karyopherin-mediated nuclear import.Elife. 2020 Mar 2;9:e51685. doi: 10.7554/eLife.51685. Elife. 2020. PMID: 32119645 Free PMC article.
-
Cytoplasmic nucleoporin assemblage: the cellular artwork in physiology and disease.Nucleus. 2024 Dec;15(1):2387534. doi: 10.1080/19491034.2024.2387534. Epub 2024 Aug 12. Nucleus. 2024. PMID: 39135336 Free PMC article. Review.
-
Remodelling of Nucleus-Vacuole Junctions During Metabolic and Proteostatic Stress.Contact (Thousand Oaks). 2021 May 27;4:25152564211016608. doi: 10.1177/25152564211016608. eCollection 2021 Jan-Dec. Contact (Thousand Oaks). 2021. PMID: 34124572 Free PMC article.
-
Structure of cytoplasmic ring of nuclear pore complex by integrative cryo-EM and AlphaFold.Science. 2022 Jun 10;376(6598):eabm9326. doi: 10.1126/science.abm9326. Epub 2022 Jun 10. Science. 2022. PMID: 35679401 Free PMC article.
References
-
- Alber F, Dokudovskaya S, Veenhoff LM, Zhang W, Kipper J, Devos D, Suprapto A, Karni-Schmidt O, Williams R, Chait BT, et al. The molecular architecture of the nuclear pore complex. Nature. 2007;450:695–701. - PubMed
-
- Allen NP, Huang L, Burlingame A, Rexach M. Proteomic analysis of nucleoporin interacting proteins. The Journal of biological chemistry. 2001;276:29268–29274. - PubMed
-
- Amlacher S, Sarges P, Flemming D, van Noort V, Kunze R, Devos DP, Arumugam M, Bork P, Hurt E. Insight into structure and assembly of the nuclear pore complex by utilizing the genome of a eukaryotic thermophile. Cell. 2011;146:277–289. - PubMed
MeSH terms
Substances
Grants and funding
LinkOut - more resources
Full Text Sources
Other Literature Sources
Molecular Biology Databases
Miscellaneous