EGFR Ligands Differentially Stabilize Receptor Dimers to Specify Signaling Kinetics
- PMID: 28988771
- PMCID: PMC5650921
- DOI: 10.1016/j.cell.2017.09.017
EGFR Ligands Differentially Stabilize Receptor Dimers to Specify Signaling Kinetics
Abstract
Epidermal growth factor receptor (EGFR) regulates many crucial cellular programs, with seven different activating ligands shaping cell signaling in distinct ways. Using crystallography and other approaches, we show how the EGFR ligands epiregulin (EREG) and epigen (EPGN) stabilize different dimeric conformations of the EGFR extracellular region. As a consequence, EREG or EPGN induce less stable EGFR dimers than EGF-making them partial agonists of EGFR dimerization. Unexpectedly, this weakened dimerization elicits more sustained EGFR signaling than seen with EGF, provoking responses in breast cancer cells associated with differentiation rather than proliferation. Our results reveal how responses to different EGFR ligands are defined by receptor dimerization strength and signaling dynamics. These findings have broad implications for understanding receptor tyrosine kinase (RTK) signaling specificity. Our results also suggest parallels between partial and/or biased agonism in RTKs and G-protein-coupled receptors, as well as new therapeutic opportunities for correcting RTK signaling output.
Keywords: biased agonist; cell fate decision; crystallography; dimerization; growth factor; kinetic proofreading; negative feedback; phosphatase; receptor tyrosine kinase; signaling specificity.
Copyright © 2017 Elsevier Inc. All rights reserved.
Figures
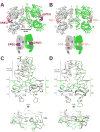
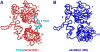
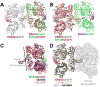
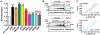
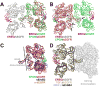
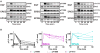
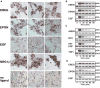
Similar articles
-
Glioblastoma mutations alter EGFR dimer structure to prevent ligand bias.Nature. 2022 Feb;602(7897):518-522. doi: 10.1038/s41586-021-04393-3. Epub 2022 Feb 9. Nature. 2022. PMID: 35140400 Free PMC article.
-
Epiregulin-blocking antibody inhibits epiregulin-dependent EGFR signaling.Biochem Biophys Res Commun. 2017 Jul 15;489(1):83-88. doi: 10.1016/j.bbrc.2017.03.006. Epub 2017 Mar 6. Biochem Biophys Res Commun. 2017. PMID: 28274874
-
Role of EGF receptor ligands in TCDD-induced EGFR down-regulation and cellular proliferation.Chem Biol Interact. 2016 Jun 25;253:38-47. doi: 10.1016/j.cbi.2016.04.031. Epub 2016 Apr 23. Chem Biol Interact. 2016. PMID: 27117977 Free PMC article.
-
EGF receptor ligands: recent advances.F1000Res. 2016 Sep 8;5:F1000 Faculty Rev-2270. doi: 10.12688/f1000research.9025.1. eCollection 2016. F1000Res. 2016. PMID: 27635238 Free PMC article. Review.
-
The ErbB/HER family of protein-tyrosine kinases and cancer.Pharmacol Res. 2014 Jan;79:34-74. doi: 10.1016/j.phrs.2013.11.002. Epub 2013 Nov 20. Pharmacol Res. 2014. PMID: 24269963 Review.
Cited by
-
Epiregulin is a dendritic cell-derived EGFR ligand that maintains skin and lung fibrosis.Sci Immunol. 2022 Dec 16;7(78):eabq6691. doi: 10.1126/sciimmunol.abq6691. Epub 2022 Dec 9. Sci Immunol. 2022. PMID: 36490328 Free PMC article.
-
Fyn and TOM1L1 are recruited to clathrin-coated pits and regulate Akt signaling.J Cell Biol. 2022 Apr 4;221(4):e201808181. doi: 10.1083/jcb.201808181. Epub 2022 Mar 3. J Cell Biol. 2022. PMID: 35238864 Free PMC article.
-
Cryo-EM analyses reveal the common mechanism and diversification in the activation of RET by different ligands.Elife. 2019 Sep 19;8:e47650. doi: 10.7554/eLife.47650. Elife. 2019. PMID: 31535977 Free PMC article.
-
Quantitative assessment of ligand bias from bias plots: The bias coefficient "kappa".Biochim Biophys Acta Gen Subj. 2023 Oct;1867(10):130428. doi: 10.1016/j.bbagen.2023.130428. Epub 2023 Jul 23. Biochim Biophys Acta Gen Subj. 2023. PMID: 37488010 Free PMC article.
-
Biased activation of the receptor tyrosine kinase HER2.Cell Mol Life Sci. 2023 May 20;80(6):158. doi: 10.1007/s00018-023-04806-8. Cell Mol Life Sci. 2023. PMID: 37208479 Free PMC article.
References
-
- Aksamitiene E, Hoek JB, Kiyatkin A. Multistrip Western blotting: a tool for comparative quantitative analysis of multiple proteins. Methods Mol Biol. 2015;1312:197–226. - PubMed
MeSH terms
Substances
Grants and funding
LinkOut - more resources
Full Text Sources
Other Literature Sources
Molecular Biology Databases
Research Materials
Miscellaneous