RUNX2 expression in thyroid and breast cancer requires the cooperation of three non-redundant enhancers under the control of BRD4 and c-JUN
- PMID: 28981843
- PMCID: PMC5737559
- DOI: 10.1093/nar/gkx802
RUNX2 expression in thyroid and breast cancer requires the cooperation of three non-redundant enhancers under the control of BRD4 and c-JUN
Abstract
Aberrant reactivation of embryonic pathways is a common feature of cancer. RUNX2 is a transcription factor crucial during embryogenesis that is aberrantly reactivated in many tumors, including thyroid and breast cancer, where it promotes aggressiveness and metastatic spreading. Currently, the mechanisms driving RUNX2 expression in cancer are still largely unknown. Here we showed that RUNX2 transcription in thyroid and breast cancer requires the cooperation of three distantly located enhancers (ENHs) brought together by chromatin three-dimensional looping. We showed that BRD4 controls RUNX2 by binding to the newly identified ENHs and we demonstrated that the anti-proliferative effects of bromodomain inhibitors (BETi) is associated with RUNX2 transcriptional repression. We demonstrated that each RUNX2 ENH is potentially controlled by a distinct set of TFs and we identified c-JUN as the principal pivot of this regulatory platform. We also observed that accumulation of genetic mutations within these elements correlates with metastatic behavior in human thyroid tumors. Finally, we identified RAINs, a novel family of ENH-associated long non-coding RNAs, transcribed from the identified RUNX2 regulatory unit. Our data provide a new model to explain how RUNX2 expression is reactivated in thyroid and breast cancer and how cancer-driving signaling pathways converge on the regulation of this gene.
© The Author(s) 2017. Published by Oxford University Press on behalf of Nucleic Acids Research.
Figures
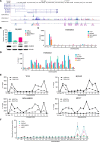
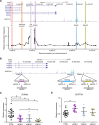
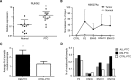
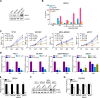
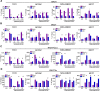
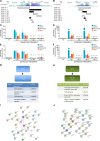
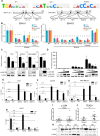
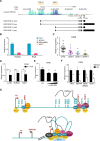
Similar articles
-
HDACs control RUNX2 expression in cancer cells through redundant and cell context-dependent mechanisms.J Exp Clin Cancer Res. 2019 Aug 8;38(1):346. doi: 10.1186/s13046-019-1350-5. J Exp Clin Cancer Res. 2019. PMID: 31395086 Free PMC article.
-
RUNX2 and TAZ-dependent signaling pathways regulate soluble E-Cadherin levels and tumorsphere formation in breast cancer cells.Oncotarget. 2015 Sep 29;6(29):28132-50. doi: 10.18632/oncotarget.4654. Oncotarget. 2015. PMID: 26320173 Free PMC article.
-
RAIN Is a Novel Enhancer-Associated lncRNA That Controls RUNX2 Expression and Promotes Breast and Thyroid Cancer.Mol Cancer Res. 2020 Jan;18(1):140-152. doi: 10.1158/1541-7786.MCR-19-0564. Epub 2019 Oct 17. Mol Cancer Res. 2020. PMID: 31624086
-
RUNX2 and the PI3K/AKT axis reciprocal activation as a driving force for tumor progression.Mol Cancer. 2015 Jul 25;14:137. doi: 10.1186/s12943-015-0404-3. Mol Cancer. 2015. PMID: 26204939 Free PMC article. Review.
-
Regulatory roles of Runx2 in metastatic tumor and cancer cell interactions with bone.Cancer Metastasis Rev. 2006 Dec;25(4):589-600. doi: 10.1007/s10555-006-9032-0. Cancer Metastasis Rev. 2006. PMID: 17165130 Review.
Cited by
-
No Need to Stick Together to Be Connected: Multiple Types of Enhancers' Networking.Cancers (Basel). 2021 Oct 16;13(20):5201. doi: 10.3390/cancers13205201. Cancers (Basel). 2021. PMID: 34680347 Free PMC article. Review.
-
The network of non-coding RNAs and their molecular targets in breast cancer.Mol Cancer. 2020 Mar 18;19(1):61. doi: 10.1186/s12943-020-01181-x. Mol Cancer. 2020. PMID: 32188472 Free PMC article. Review.
-
Significance of LINC00460 in the progression and prognosis in digestive tract tumors.Zhong Nan Da Xue Xue Bao Yi Xue Ban. 2021 Jun 28;46(6):628-636. doi: 10.11817/j.issn.1672-7347.2021.200975. Zhong Nan Da Xue Xue Bao Yi Xue Ban. 2021. PMID: 34275932 Free PMC article. Chinese, English.
-
RUNX Proteins as Epigenetic Modulators in Cancer.Cells. 2022 Nov 20;11(22):3687. doi: 10.3390/cells11223687. Cells. 2022. PMID: 36429115 Free PMC article. Review.
-
The Hippo pathway modulates resistance to BET proteins inhibitors in lung cancer cells.Oncogene. 2019 Oct;38(42):6801-6817. doi: 10.1038/s41388-019-0924-1. Epub 2019 Aug 12. Oncogene. 2019. PMID: 31406246
References
MeSH terms
Substances
LinkOut - more resources
Full Text Sources
Other Literature Sources
Research Materials
Miscellaneous