Commensal Propionibacterium strain UF1 mitigates intestinal inflammation via Th17 cell regulation
- PMID: 28945202
- PMCID: PMC5663347
- DOI: 10.1172/JCI95376
Commensal Propionibacterium strain UF1 mitigates intestinal inflammation via Th17 cell regulation
Abstract
Consumption of human breast milk (HBM) attenuates the incidence of necrotizing enterocolitis (NEC), which remains a leading and intractable cause of mortality in preterm infants. Here, we report that this diminution correlates with alterations in the gut microbiota, particularly enrichment of Propionibacterium species. Transfaunation of microbiota from HBM-fed preterm infants or a newly identified and cultured Propionibacterium strain, P. UF1, to germfree mice conferred protection against pathogen infection and correlated with profound increases in intestinal Th17 cells. The induction of Th17 cells was dependent on bacterial dihydrolipoamide acetyltransferase (DlaT), a major protein expressed on the P. UF1 surface layer (S-layer). Binding of P. UF1 to its cognate receptor, SIGNR1, on dendritic cells resulted in the regulation of intestinal phagocytes. Importantly, transfer of P. UF1 profoundly mitigated induced NEC-like injury in neonatal mice. Together, these results mechanistically elucidate the protective effects of HBM and P. UF1-induced immunoregulation, which safeguard against proinflammatory diseases, including NEC.
Conflict of interest statement
Figures
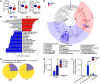
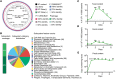
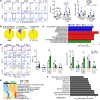
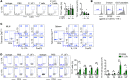
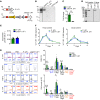
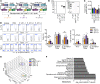
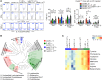
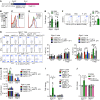
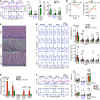
Similar articles
-
Regulation of Th17 cells by P. UF1 against systemic Listeria monocytogenes infection.Gut Microbes. 2018;9(3):279-287. doi: 10.1080/19490976.2017.1417731. Epub 2018 Feb 8. Gut Microbes. 2018. PMID: 29420115 Free PMC article.
-
Neonatal intestinal immune regulation by the commensal bacterium, P. UF1.Mucosal Immunol. 2019 Mar;12(2):434-444. doi: 10.1038/s41385-018-0125-1. Epub 2019 Jan 15. Mucosal Immunol. 2019. PMID: 30647410 Free PMC article.
-
Regulating colonic dendritic cells by commensal glycosylated large surface layer protein A to sustain gut homeostasis against pathogenic inflammation.Mucosal Immunol. 2020 Jan;13(1):34-46. doi: 10.1038/s41385-019-0210-0. Epub 2019 Oct 16. Mucosal Immunol. 2020. PMID: 31619761 Free PMC article.
-
Necrotizing Enterocolitis and the Preterm Infant Microbiome.Adv Exp Med Biol. 2019;1125:25-36. doi: 10.1007/5584_2018_313. Adv Exp Med Biol. 2019. PMID: 30680646 Review.
-
Pathogenesis of necrotising enterocolitis: The impact of the altered gut microbiota and antibiotic exposure in preterm infants.Acta Paediatr. 2021 Feb;110(2):433-440. doi: 10.1111/apa.15559. Epub 2020 Sep 28. Acta Paediatr. 2021. PMID: 32876963 Review.
Cited by
-
Early gut microbiota intervention in premature infants: Application perspectives.J Adv Res. 2023 Sep;51:59-72. doi: 10.1016/j.jare.2022.11.004. Epub 2022 Nov 11. J Adv Res. 2023. PMID: 36372205 Free PMC article. Review.
-
Effect of the Microbiome on Intestinal Innate Immune Development in Early Life and the Potential Strategy of Early Intervention.Front Immunol. 2022 Jul 19;13:936300. doi: 10.3389/fimmu.2022.936300. eCollection 2022. Front Immunol. 2022. PMID: 35928828 Free PMC article. Review.
-
Review: Adaptation of Beneficial Propionibacteria, Lactobacilli, and Bifidobacteria Improves Tolerance Toward Technological and Digestive Stresses.Front Microbiol. 2019 Apr 24;10:841. doi: 10.3389/fmicb.2019.00841. eCollection 2019. Front Microbiol. 2019. PMID: 31068918 Free PMC article. Review.
-
Short-Term Tomato Consumption Alters the Pig Gut Microbiome toward a More Favorable Profile.Microbiol Spectr. 2022 Dec 21;10(6):e0250622. doi: 10.1128/spectrum.02506-22. Epub 2022 Nov 8. Microbiol Spectr. 2022. PMID: 36346230 Free PMC article.
-
Effect of diet on pathogen performance in the microbiome.Microbiome Res Rep. 2022 Mar 26;1(2):13. doi: 10.20517/mrr.2021.10. eCollection 2022. Microbiome Res Rep. 2022. PMID: 38045644 Free PMC article. Review.
References
MeSH terms
Substances
Grants and funding
LinkOut - more resources
Full Text Sources
Other Literature Sources