N6-methyladenosine (m6A) recruits and repels proteins to regulate mRNA homeostasis
- PMID: 28869609
- PMCID: PMC5725193
- DOI: 10.1038/nsmb.3462
N6-methyladenosine (m6A) recruits and repels proteins to regulate mRNA homeostasis
Abstract
RNA modifications are integral to the regulation of RNA metabolism. One abundant mRNA modification is N6-methyladenosine (m6A), which affects various aspects of RNA metabolism, including splicing, translation and degradation. Current knowledge about the proteins recruited to m6A to carry out these molecular processes is still limited. Here we describe comprehensive and systematic mass-spectrometry-based screening of m6A interactors in various cell types and sequence contexts. Among the main findings, we identified G3BP1 as a protein that is repelled by m6A and positively regulates mRNA stability in an m6A-regulated manner. Furthermore, we identified FMR1 as a sequence-context-dependent m6A reader, thus revealing a connection between an mRNA modification and an autism spectrum disorder. Collectively, our data represent a rich resource and shed further light on the complex interplay among m6A, m6A interactors and mRNA homeostasis.
Conflict of interest statement
The authors declare no competing financial interests.
Figures
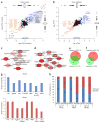
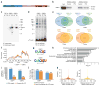
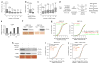
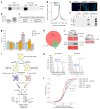
Similar articles
-
Structural Insights into N6-methyladenosine (m6A) Modification in the Transcriptome.Genomics Proteomics Bioinformatics. 2018 Apr;16(2):85-98. doi: 10.1016/j.gpb.2018.03.001. Epub 2018 Apr 27. Genomics Proteomics Bioinformatics. 2018. PMID: 29709557 Free PMC article. Review.
-
N(6)-methyladenosine Modulates Messenger RNA Translation Efficiency.Cell. 2015 Jun 4;161(6):1388-99. doi: 10.1016/j.cell.2015.05.014. Cell. 2015. PMID: 26046440 Free PMC article.
-
The m6A-methylase complex recruits TREX and regulates mRNA export.Sci Rep. 2018 Sep 14;8(1):13827. doi: 10.1038/s41598-018-32310-8. Sci Rep. 2018. PMID: 30218090 Free PMC article.
-
m(6)A: Signaling for mRNA splicing.RNA Biol. 2016 Sep;13(9):756-9. doi: 10.1080/15476286.2016.1201628. Epub 2016 Jun 28. RNA Biol. 2016. PMID: 27351695 Free PMC article. Review.
-
[Progress in epigenetic modification of mRNA and the function of m6A modification].Sheng Wu Gong Cheng Xue Bao. 2019 May 25;35(5):775-783. doi: 10.13345/j.cjb.180416. Sheng Wu Gong Cheng Xue Bao. 2019. PMID: 31222996 Review. Chinese.
Cited by
-
Potential roles of N6-methyladenosine (m6A) in immune cells.J Transl Med. 2021 Jun 8;19(1):251. doi: 10.1186/s12967-021-02918-y. J Transl Med. 2021. PMID: 34103054 Free PMC article. Review.
-
Genetic analyses support the contribution of mRNA N6-methyladenosine (m6A) modification to human disease heritability.Nat Genet. 2020 Sep;52(9):939-949. doi: 10.1038/s41588-020-0644-z. Epub 2020 Jun 29. Nat Genet. 2020. PMID: 32601472 Free PMC article.
-
Emerging Mutual Regulatory Roles between m6A Modification and microRNAs.Int J Mol Sci. 2023 Jan 1;24(1):773. doi: 10.3390/ijms24010773. Int J Mol Sci. 2023. PMID: 36614216 Free PMC article. Review.
-
N6-Methyladenosine Modification Controls Circular RNA Immunity.Mol Cell. 2019 Oct 3;76(1):96-109.e9. doi: 10.1016/j.molcel.2019.07.016. Epub 2019 Aug 29. Mol Cell. 2019. PMID: 31474572 Free PMC article.
-
RNA modifications modulate gene expression during development.Science. 2018 Sep 28;361(6409):1346-1349. doi: 10.1126/science.aau1646. Science. 2018. PMID: 30262497 Free PMC article. Review.
References
-
- Dimock K, Stoltzfus CM. Sequence specificity of internal methylation in B77 avian sarcoma virus RNA subunits. Biochemistry. 1977;16:471–478. - PubMed
-
- Desrosiers RC, Friderici KH, Rottman FM. Characterization of Novikoff hepatoma mRNA methylation and heterogeneity in the methylated 5′ terminus. Biochemistry. 1975;14:4367–4374. - PubMed
MeSH terms
Substances
Grants and funding
LinkOut - more resources
Full Text Sources
Other Literature Sources
Molecular Biology Databases
Research Materials
Miscellaneous