Chemokines encoded by herpesviruses
- PMID: 28848041
- PMCID: PMC5638632
- DOI: 10.1189/jlb.4RU0417-145RR
Chemokines encoded by herpesviruses
Abstract
Viruses use diverse strategies to elude the immune system, including copying and repurposing host cytokine and cytokine receptor genes. For herpesviruses, the chemokine system of chemotactic cytokines and receptors is a common source of copied genes. Here, we review the current state of knowledge about herpesvirus-encoded chemokines and discuss their possible roles in viral pathogenesis, as well as their clinical potential as novel anti-inflammatory agents or targets for new antiviral strategies.
Keywords: Chemotaxis; chemokine receptors; immune evasion; viral chemokine.
© Society for Leukocyte Biology.
Figures
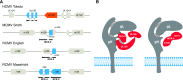
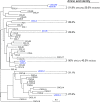
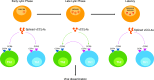
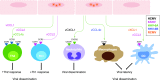
Similar articles
-
Chemokine Subversion by Human Herpesviruses.J Innate Immun. 2018;10(5-6):465-478. doi: 10.1159/000492161. Epub 2018 Aug 30. J Innate Immun. 2018. PMID: 30165356 Free PMC article. Review.
-
Piracy on the molecular level: human herpesviruses manipulate cellular chemotaxis.J Gen Virol. 2016 Mar;97(3):543-560. doi: 10.1099/jgv.0.000370. Epub 2015 Dec 14. J Gen Virol. 2016. PMID: 26669819 Review.
-
[Modulation of immune system by virus infection].Rinsho Byori. 2006 Feb;54(2):159-69. Rinsho Byori. 2006. PMID: 16548237 Review. Japanese.
-
Virally encoded 7TM receptors.Oncogene. 2001 Mar 26;20(13):1582-93. doi: 10.1038/sj.onc.1204191. Oncogene. 2001. PMID: 11313905 Review.
-
[Herpesvirus infection].Nihon Rinsho. 2005 Apr;63 Suppl 4:495-502. Nihon Rinsho. 2005. PMID: 15861701 Review. Japanese. No abstract available.
Cited by
-
KSHV: Immune Modulation and Immunotherapy.Front Immunol. 2020 Feb 7;10:3084. doi: 10.3389/fimmu.2019.03084. eCollection 2019. Front Immunol. 2020. PMID: 32117196 Free PMC article. Review.
-
The chemokine receptor CCR5: multi-faceted hook for HIV-1.Retrovirology. 2024 Jan 23;21(1):2. doi: 10.1186/s12977-024-00634-1. Retrovirology. 2024. PMID: 38263120 Free PMC article. Review.
-
Chemokine Subversion by Human Herpesviruses.J Innate Immun. 2018;10(5-6):465-478. doi: 10.1159/000492161. Epub 2018 Aug 30. J Innate Immun. 2018. PMID: 30165356 Free PMC article. Review.
-
Regulation and Function of Chemokines at the Maternal-Fetal Interface.Front Cell Dev Biol. 2022 Jul 22;10:826053. doi: 10.3389/fcell.2022.826053. eCollection 2022. Front Cell Dev Biol. 2022. PMID: 35938162 Free PMC article. Review.
-
Today's Kaposi sarcoma is not the same as it was 40 years ago, or is it?J Med Virol. 2023 May;95(5):e28773. doi: 10.1002/jmv.28773. J Med Virol. 2023. PMID: 37212317 Free PMC article. Review.
References
-
- Arvin A., Campadelli-Fiume G., Mocarski E., Moore P. S., Roizman B., Whitley R., Yamanishi K., eds. (2007) Human Herpesviruses: Biology, Therapy, and Immunoprophylaxis, Cambridge University Press, Cambridge. - PubMed
-
- Bloom D. C. (2016) Alphaherpesvirus latency: a dynamic state of transcription and reactivation. Adv. Virus Res. 94, 53–80. - PubMed
-
- Ressing M. E., van Gent M., Gram A. M., Hooykaas M. J.., Piersma S. J., Wiertz E. J. (2015) Immune evasion by Epstein-Barr virus. Curr. Top. Microbiol. Immunol. 391, 355–381. - PubMed
-
- Grey F. (2015) Role of microRNAs in herpesvirus latency and persistence. J. Gen. Virol. 96, 739–751. - PubMed
Publication types
MeSH terms
Substances
LinkOut - more resources
Full Text Sources
Other Literature Sources