Targeting cellular senescence prevents age-related bone loss in mice
- PMID: 28825716
- PMCID: PMC5657592
- DOI: 10.1038/nm.4385
Targeting cellular senescence prevents age-related bone loss in mice
Erratum in
-
Corrigendum: Targeting cellular senescence prevents age-related bone loss in mice.Nat Med. 2017 Nov 7;23(11):1384. doi: 10.1038/nm1117-1384c. Nat Med. 2017. PMID: 29117174
Abstract
Aging is associated with increased cellular senescence, which is hypothesized to drive the eventual development of multiple comorbidities. Here we investigate a role for senescent cells in age-related bone loss through multiple approaches. In particular, we used either genetic (i.e., the INK-ATTAC 'suicide' transgene encoding an inducible caspase 8 expressed specifically in senescent cells) or pharmacological (i.e., 'senolytic' compounds) means to eliminate senescent cells. We also inhibited the production of the proinflammatory secretome of senescent cells using a JAK inhibitor (JAKi). In aged (20- to 22-month-old) mice with established bone loss, activation of the INK-ATTAC caspase 8 in senescent cells or treatment with senolytics or the JAKi for 2-4 months resulted in higher bone mass and strength and better bone microarchitecture than in vehicle-treated mice. The beneficial effects of targeting senescent cells were due to lower bone resorption with either maintained (trabecular) or higher (cortical) bone formation as compared to vehicle-treated mice. In vitro studies demonstrated that senescent-cell conditioned medium impaired osteoblast mineralization and enhanced osteoclast-progenitor survival, leading to increased osteoclastogenesis. Collectively, these data establish a causal role for senescent cells in bone loss with aging, and demonstrate that targeting these cells has both anti-resorptive and anabolic effects on bone. Given that eliminating senescent cells and/or inhibiting their proinflammatory secretome also improves cardiovascular function, enhances insulin sensitivity, and reduces frailty, targeting this fundamental mechanism to prevent age-related bone loss suggests a novel treatment strategy not only for osteoporosis, but also for multiple age-related comorbidities.
Conflict of interest statement
Figures
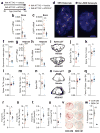
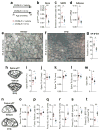
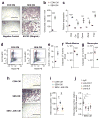
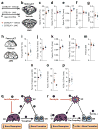
Comment in
-
Bone: Targeting old cells to protect old bones.Nat Rev Rheumatol. 2017 Nov;13(11):632. doi: 10.1038/nrrheum.2017.152. Epub 2017 Sep 14. Nat Rev Rheumatol. 2017. PMID: 28905853 No abstract available.
-
Diabetes: New marker to predict risk of T2DM.Nat Rev Endocrinol. 2017 Nov;13(11):625. doi: 10.1038/nrendo.2017.128. Epub 2017 Sep 22. Nat Rev Endocrinol. 2017. PMID: 28937688 No abstract available.
Similar articles
-
Independent Roles of Estrogen Deficiency and Cellular Senescence in the Pathogenesis of Osteoporosis: Evidence in Young Adult Mice and Older Humans.J Bone Miner Res. 2019 Aug;34(8):1407-1418. doi: 10.1002/jbmr.3729. Epub 2019 Jun 21. J Bone Miner Res. 2019. PMID: 30913313 Free PMC article.
-
Elimination of senescent osteoclast progenitors has no effect on the age-associated loss of bone mass in mice.Aging Cell. 2019 Jun;18(3):e12923. doi: 10.1111/acel.12923. Epub 2019 Feb 17. Aging Cell. 2019. PMID: 30773784 Free PMC article.
-
Identification of Senescent Cells in the Bone Microenvironment.J Bone Miner Res. 2016 Nov;31(11):1920-1929. doi: 10.1002/jbmr.2892. Epub 2016 Oct 24. J Bone Miner Res. 2016. PMID: 27341653 Free PMC article.
-
Cellular senescence in bone.Bone. 2019 Apr;121:121-133. doi: 10.1016/j.bone.2019.01.015. Epub 2019 Jan 16. Bone. 2019. PMID: 30659978 Free PMC article. Review.
-
Inhibiting Cellular Senescence: A New Therapeutic Paradigm for Age-Related Osteoporosis.J Clin Endocrinol Metab. 2018 Apr 1;103(4):1282-1290. doi: 10.1210/jc.2017-02694. J Clin Endocrinol Metab. 2018. PMID: 29425296 Free PMC article. Review.
Cited by
-
Targeting cellular senescence in cancer and aging: roles of p53 and its isoforms.Carcinogenesis. 2020 Aug 12;41(8):1017-1029. doi: 10.1093/carcin/bgaa071. Carcinogenesis. 2020. PMID: 32619002 Free PMC article. Review.
-
Augmentation of Bone Regeneration by Depletion of Stress-Induced Senescent Cells Using Catechin and Senolytics.Int J Mol Sci. 2020 Jun 13;21(12):4213. doi: 10.3390/ijms21124213. Int J Mol Sci. 2020. PMID: 32545756 Free PMC article.
-
The Role of Methionine-Rich Diet in Unhealthy Cerebrovascular and Brain Aging: Mechanisms and Implications for Cognitive Impairment.Nutrients. 2023 Nov 3;15(21):4662. doi: 10.3390/nu15214662. Nutrients. 2023. PMID: 37960316 Free PMC article. Review.
-
Cellular senescence is a key mediator of lung aging and susceptibility to infection.Front Immunol. 2022 Aug 31;13:1006710. doi: 10.3389/fimmu.2022.1006710. eCollection 2022. Front Immunol. 2022. PMID: 36119079 Free PMC article. Review.
-
Effects and Mechanisms of Five Psoralea Prenylflavonoids on Aging-Related Diseases.Oxid Med Cell Longev. 2020 Jun 17;2020:2128513. doi: 10.1155/2020/2128513. eCollection 2020. Oxid Med Cell Longev. 2020. PMID: 32655760 Free PMC article. Review.
References
MeSH terms
Substances
Grants and funding
LinkOut - more resources
Full Text Sources
Other Literature Sources
Medical
Molecular Biology Databases