Primary structure and expression of a functional human glucocorticoid receptor cDNA
- PMID: 2867473
- PMCID: PMC6165583
- DOI: 10.1038/318635a0
Primary structure and expression of a functional human glucocorticoid receptor cDNA
Abstract
Identification of complementary DNAs encoding the human glucocorticoid receptor predicts two protein forms, of 777 (alpha) and 742 (beta) amino acids, which differ at their carboxy termini. The proteins contain a cysteine/lysine/arginine-rich region which may define the DNA-binding domain. Pure radiolabelled glucocorticoid receptor, synthesized in vitro, is immunoreactive and possesses intrinsic steroid-binding activity characteristic of the native glucocorticoid receptor.
Figures
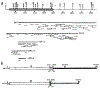
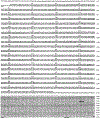
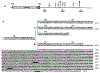
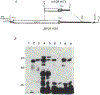
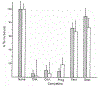
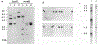
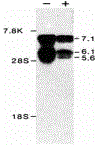
Similar articles
-
Domain structure of human glucocorticoid receptor and its relationship to the v-erb-A oncogene product.Nature. 1985 Dec 19-1986 Jan 1;318(6047):670-2. doi: 10.1038/318670a0. Nature. 1985. PMID: 3841189
-
Human steroid receptors and erb-A gene products form a superfamily of enhancer-binding proteins.Clin Physiol Biochem. 1987;5(3-4):179-89. Clin Physiol Biochem. 1987. PMID: 3304776
-
Intragenic sequences of the human glucocorticoid receptor complementary DNA mediate hormone-inducible receptor messenger RNA down-regulation through multiple mechanisms.Mol Endocrinol. 1994 Dec;8(12):1764-73. doi: 10.1210/mend.8.12.7708063. Mol Endocrinol. 1994. PMID: 7708063
-
DNA-binding by the glucocorticoid receptor: a structural and functional analysis.J Steroid Biochem Mol Biol. 1992 Mar;41(3-8):249-72. doi: 10.1016/0960-0760(92)90351-i. J Steroid Biochem Mol Biol. 1992. PMID: 1562506 Review.
-
The structure of the human glucocorticoid receptor and its gene.J Steroid Biochem. 1987;27(1-3):105-8. doi: 10.1016/0022-4731(87)90300-1. J Steroid Biochem. 1987. PMID: 2447387 Review.
Cited by
-
Early onset pre-eclampsia is associated with altered DNA methylation of cortisol-signalling and steroidogenic genes in the placenta.PLoS One. 2013 May 7;8(5):e62969. doi: 10.1371/journal.pone.0062969. Print 2013. PLoS One. 2013. PMID: 23667551 Free PMC article.
-
Signaling by nuclear receptors.Cold Spring Harb Perspect Biol. 2013 Mar 1;5(3):a016709. doi: 10.1101/cshperspect.a016709. Cold Spring Harb Perspect Biol. 2013. PMID: 23457262 Free PMC article. Review.
-
Obestatin signalling counteracts glucocorticoid-induced skeletal muscle atrophy via NEDD4/KLF15 axis.J Cachexia Sarcopenia Muscle. 2021 Apr;12(2):493-505. doi: 10.1002/jcsm.12677. Epub 2021 Mar 9. J Cachexia Sarcopenia Muscle. 2021. PMID: 33687156 Free PMC article.
-
Deletion mapping of DNA markers to a region of chromosome 5 that cosegregates with schizophrenia.Genomics. 1989 Nov;5(4):940-4. doi: 10.1016/0888-7543(89)90138-9. Genomics. 1989. PMID: 2591972 Free PMC article.
-
Differential regulation by dexamethasone of glucocorticoid receptor messenger RNA concentrations in neuronal cultures derived from fetal rat hypothalamus and cerebral cortex.Cell Mol Neurobiol. 1990 Jun;10(2):227-35. doi: 10.1007/BF00734576. Cell Mol Neurobiol. 1990. PMID: 2364410
References
-
- Jensen EV & De Sombre ER A. Rev. Biochem 41, 203–230 (1972). - PubMed
-
- Gorski J & Gannon FA, Rev. Physiol 38, 425–450 (1976). - PubMed
-
- Yamamoto K,R & Alberts BM A. Rev. Biochem 45, 721–746 (1976). - PubMed
-
- O’Malley BW, McGuire WL, Kohler PO & Komtman SG Recent Prog. Horm. Res 25, 105–160 (1969). - PubMed
Publication types
MeSH terms
Substances
Associated data
- Actions
- Actions
Grants and funding
LinkOut - more resources
Full Text Sources
Other Literature Sources
Molecular Biology Databases
Research Materials