Dedifferentiation, Proliferation, and Redifferentiation of Adult Mammalian Cardiomyocytes After Ischemic Injury
- PMID: 28642276
- PMCID: PMC5575972
- DOI: 10.1161/CIRCULATIONAHA.116.024307
Dedifferentiation, Proliferation, and Redifferentiation of Adult Mammalian Cardiomyocytes After Ischemic Injury
Abstract
Background: Adult mammalian hearts have a limited ability to generate new cardiomyocytes. Proliferation of existing adult cardiomyocytes (ACMs) is a potential source of new cardiomyocytes. Understanding the fundamental biology of ACM proliferation could be of great clinical significance for treating myocardial infarction (MI). We aim to understand the process and regulation of ACM proliferation and its role in new cardiomyocyte formation of post-MI mouse hearts.
Methods: β-Actin-green fluorescent protein transgenic mice and fate-mapping Myh6-MerCreMer-tdTomato/lacZ mice were used to trace the fate of ACMs. In a coculture system with neonatal rat ventricular myocytes, ACM proliferation was documented with clear evidence of cytokinesis observed with time-lapse imaging. Cardiomyocyte proliferation in the adult mouse post-MI heart was detected by cell cycle markers and 5-ethynyl-2-deoxyuridine incorporation analysis. Echocardiography was used to measure cardiac function, and histology was performed to determine infarction size.
Results: In vitro, mononucleated and bi/multinucleated ACMs were able to proliferate at a similar rate (7.0%) in the coculture. Dedifferentiation proceeded ACM proliferation, which was followed by redifferentiation. Redifferentiation was essential to endow the daughter cells with cardiomyocyte contractile function. Intercellular propagation of Ca2+ from contracting neonatal rat ventricular myocytes into ACM daughter cells was required to activate the Ca2+-dependent calcineurin-nuclear factor of activated T-cell signaling pathway to induce ACM redifferentiation. The properties of neonatal rat ventricular myocyte Ca2+ transients influenced the rate of ACM redifferentiation. Hypoxia impaired the function of gap junctions by dephosphorylating its component protein connexin 43, the major mediator of intercellular Ca2+ propagation between cardiomyocytes, thereby impairing ACM redifferentiation. In vivo, ACM proliferation was found primarily in the MI border zone. An ischemia-resistant connexin 43 mutant enhanced the redifferentiation of ACM-derived new cardiomyocytes after MI and improved cardiac function.
Conclusions: Mature ACMs can reenter the cell cycle and form new cardiomyocytes through a 3-step process: dedifferentiation, proliferation, and redifferentiation. Intercellular Ca2+ signal from neighboring functioning cardiomyocytes through gap junctions induces the redifferentiation process. This novel mechanism contributes to new cardiomyocyte formation in post-MI hearts in mammals.
Keywords: cell dedifferentiation; cellular proliferation; myocardial infarction; myocytes, cardiac; redifferentiation.
© 2017 American Heart Association, Inc.
Figures
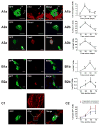
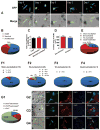
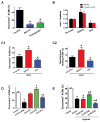
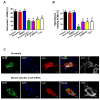
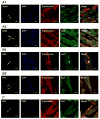
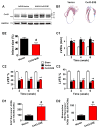
Comment in
-
Basic research: New cardiomyocyte formation in adult mouse hearts.Nat Rev Cardiol. 2017 Sep;14(9):505. doi: 10.1038/nrcardio.2017.109. Epub 2017 Jul 13. Nat Rev Cardiol. 2017. PMID: 28703224 No abstract available.
Similar articles
-
Overexpression of Tbx20 in Adult Cardiomyocytes Promotes Proliferation and Improves Cardiac Function After Myocardial Infarction.Circulation. 2016 Mar 15;133(11):1081-92. doi: 10.1161/CIRCULATIONAHA.115.019357. Epub 2016 Feb 3. Circulation. 2016. PMID: 26841808 Free PMC article.
-
Survival and maturation of human embryonic stem cell-derived cardiomyocytes in rat hearts.J Mol Cell Cardiol. 2007 Oct;43(4):504-16. doi: 10.1016/j.yjmcc.2007.07.001. Epub 2007 Jul 14. J Mol Cell Cardiol. 2007. PMID: 17707399 Free PMC article.
-
Loss of Endogenously Cycling Adult Cardiomyocytes Worsens Myocardial Function.Circ Res. 2021 Jan 22;128(2):155-168. doi: 10.1161/CIRCRESAHA.120.318277. Epub 2020 Nov 4. Circ Res. 2021. PMID: 33146578
-
Interventions in WNT Signaling to Induce Cardiomyocyte Proliferation: Crosstalk with Other Pathways.Mol Pharmacol. 2020 Feb;97(2):90-101. doi: 10.1124/mol.119.118018. Epub 2019 Nov 22. Mol Pharmacol. 2020. PMID: 31757861 Review.
-
Atypically Shaped Cardiomyocytes (ACMs): The Identification, Characterization and New Insights into a Subpopulation of Cardiomyocytes.Biomolecules. 2022 Jun 27;12(7):896. doi: 10.3390/biom12070896. Biomolecules. 2022. PMID: 35883452 Free PMC article. Review.
Cited by
-
Promoting cardiomyocyte proliferation for myocardial regeneration in large mammals.J Mol Cell Cardiol. 2024 Mar;188:52-60. doi: 10.1016/j.yjmcc.2024.01.005. Epub 2024 Feb 9. J Mol Cell Cardiol. 2024. PMID: 38340541 Review.
-
Resident macrophages as potential therapeutic targets for cardiac ageing and injury.Clin Transl Immunology. 2020 Aug 26;9(8):e1167. doi: 10.1002/cti2.1167. eCollection 2020. Clin Transl Immunology. 2020. PMID: 32874584 Free PMC article. Review.
-
The effects of rosiglitazone on the neonatal rat cardiomyocyte transcriptome: a temporal analysis.Pharmacogenomics. 2019 Nov;20(16):1125-1141. doi: 10.2217/pgs-2019-0077. Pharmacogenomics. 2019. PMID: 31755367 Free PMC article.
-
Cryo-fluorescence micro-optical sectioning tomography for volumetric imaging of various whole organs with subcellular resolution.iScience. 2022 Jul 20;25(8):104805. doi: 10.1016/j.isci.2022.104805. eCollection 2022 Aug 19. iScience. 2022. PMID: 35992061 Free PMC article.
-
Exosomes from Suxiao Jiuxin pill-treated cardiac mesenchymal stem cells decrease H3K27 demethylase UTX expression in mouse cardiomyocytes in vitro.Acta Pharmacol Sin. 2018 Apr;39(4):579-586. doi: 10.1038/aps.2018.18. Epub 2018 Mar 15. Acta Pharmacol Sin. 2018. PMID: 29542684 Free PMC article.
References
-
- Alkass K, Panula J, Westman M, Wu TD, Guerquin-Kern JL, Bergmann O. No evidence for cardiomyocyte number expansion in preadolescent mice. Cell. 2015;163:1026–1036. - PubMed
MeSH terms
Substances
Grants and funding
LinkOut - more resources
Full Text Sources
Other Literature Sources
Medical
Miscellaneous