Kinesin Motor Enzymology: Chemistry, Structure, and Physics of Nanoscale Molecular Machines
- PMID: 28510227
- PMCID: PMC5418420
- DOI: 10.1007/s12551-014-0150-6
Kinesin Motor Enzymology: Chemistry, Structure, and Physics of Nanoscale Molecular Machines
Abstract
Molecular motors are enzymes that convert chemical potential energy into controlled kinetic energy for mechanical work inside cells. Understanding the biophysics of these motors is essential for appreciating life as well as apprehending diseases that arise from motor malfunction. This review focuses on kinesin motor enzymology with special emphasis on the literature that reports the chemistry, structure and physics of several different kinesin superfamily members.
Keywords: Chemical models; Enzymes; Kinesin motor enzymology; Kinesin superfamily; Molecular motors.
Figures
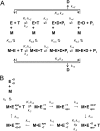
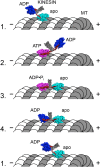
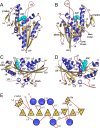
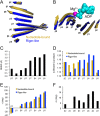
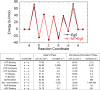
Similar articles
-
Kinesin motors as molecular machines.Bioessays. 2003 Dec;25(12):1212-9. doi: 10.1002/bies.10358. Bioessays. 2003. PMID: 14635256 Review.
-
Molecular Assembly of Rotary and Linear Motor Proteins.Acc Chem Res. 2019 Jun 18;52(6):1623-1631. doi: 10.1021/acs.accounts.9b00015. Epub 2019 Mar 18. Acc Chem Res. 2019. PMID: 30882207
-
Collective dynamics of kinesin.Phys Rev E Stat Nonlin Soft Matter Phys. 2009 Mar;79(3 Pt 1):031929. doi: 10.1103/PhysRevE.79.031929. Epub 2009 Mar 31. Phys Rev E Stat Nonlin Soft Matter Phys. 2009. PMID: 19391993
-
Subunits interactions in kinesin motors.Eur J Cell Biol. 2007 Sep;86(9):559-68. doi: 10.1016/j.ejcb.2007.05.008. Epub 2007 Jul 12. Eur J Cell Biol. 2007. PMID: 17628208 Review.
-
Experimental and theoretical energetics of walking molecular motors under fluctuating environments.Biophys Rev. 2020 Apr;12(2):503-510. doi: 10.1007/s12551-020-00684-7. Epub 2020 Mar 16. Biophys Rev. 2020. PMID: 32173796 Free PMC article. Review.
Cited by
-
Shaft Function of Kinesin-1's α4 Helix in the Processive Movement.Cell Mol Bioeng. 2019 Jun 25;12(4):345-354. doi: 10.1007/s12195-019-00581-4. eCollection 2019 Aug. Cell Mol Bioeng. 2019. PMID: 31719918 Free PMC article.
-
Sequences in the stalk domain regulate auto-inhibition and ciliary tip localization of the immotile kinesin-4 KIF7.J Cell Sci. 2021 Jul 1;134(13):jcs258464. doi: 10.1242/jcs.258464. Epub 2021 Jul 8. J Cell Sci. 2021. PMID: 34114033 Free PMC article.
-
Comparison of explicit and mean-field models of cytoskeletal filaments with crosslinking motors.Eur Phys J E Soft Matter. 2021 Mar 29;44(3):45. doi: 10.1140/epje/s10189-021-00042-9. Eur Phys J E Soft Matter. 2021. PMID: 33779863 Free PMC article.
-
How Kinesin-1 Utilize the Energy of Nucleotide: The Conformational Changes and Mechanochemical Coupling in the Unidirectional Motion of Kinesin-1.Int J Mol Sci. 2020 Sep 22;21(18):6977. doi: 10.3390/ijms21186977. Int J Mol Sci. 2020. PMID: 32972035 Free PMC article. Review.
-
New insights into the mechanochemical coupling mechanism of kinesin-microtubule complexes from their high-resolution structures.Biochem Soc Trans. 2023 Aug 31;51(4):1505-1520. doi: 10.1042/BST20221238. Biochem Soc Trans. 2023. PMID: 37560910 Free PMC article. Review.
References
-
- Admiraal SJ, Herschlag D. Mapping the transition state for ATP hydrolysis: implications for enzymatic catalysis. Chem Biol. 1995;2:729–739. - PubMed
-
- Arnal I, Metoz F, DeBonis S, Wade RH. Three-dimensional structure of functional motor proteins on microtubules. Curr Biol. 1996;6:1265–1270. - PubMed
-
- Asenjo AB, Chatterjee C, Tan D, et al (2013) Structural model for tubulin recognition and deformation by kinesin-13 microtubule depolymerases. Cell Rep 3:759–768. doi:10.1016/j.celrep.2013.01.030 - PubMed
LinkOut - more resources
Full Text Sources
Other Literature Sources