Capsular polysaccharide inhibits adhesion of Bifidobacterium longum 105-A to enterocyte-like Caco-2 cells and phagocytosis by macrophages
- PMID: 28469711
- PMCID: PMC5412050
- DOI: 10.1186/s13099-017-0177-x
Capsular polysaccharide inhibits adhesion of Bifidobacterium longum 105-A to enterocyte-like Caco-2 cells and phagocytosis by macrophages
Abstract
Background: Bifidobacterium longum 105-A produces markedly high amounts of capsular polysaccharides (CPS) and exopolysaccharides (EPS) that should play distinct roles in bacterial-host interactions. To identify the biological function of B. longum 105-A CPS/EPS, we carried out an informatics survey of the genome and identified the EPS-encoding genetic locus of B. longum 105-A that is responsible for the production of CPS/EPS. The role of CPS/EPS in the adaptation to gut tract environment and bacteria-gut cell interactions was investigated using the ΔcpsD mutant.
Results: A putative B. longum 105-A CPS/EPS gene cluster was shown to consist of 24 putative genes encoding a priming glycosyltransferase (cpsD), 7 glycosyltransferases, 4 CPS/EPS synthesis machinery proteins, and 3 dTDP-L-rhamnose synthesis enzymes. These enzymes should form a complex system that is involved in the biogenesis of CPS and/or EPS. To confirm this, we constructed a knockout mutant (ΔcpsD) by a double cross-over homologous recombination. Compared to wild-type, the ∆cpsD mutant showed a similar growth rate. However, it showed quicker sedimentation and formation of cell clusters in liquid culture. EPS was secreted by the ∆cpsD mutant, but had altered monosaccharide composition and molecular weight. Comparison of the morphology of B. longum 105-A wild-type and ∆cpsD by negative staining in light and electron microscopy revealed that the formation of fimbriae is drastically enhanced in the ∆cpsD mutant while the B. longum 105-A wild-type was coated by a thick capsule. The fimbriae expression in the ∆cpsD was closely associated with the disappearance of the CPS layer. The wild-type showed low pH tolerance, adaptation, and bile salt tolerance, but the ∆cpsD mutant had lost this survivability in gastric and duodenal environments. The ∆cpsD mutant was extensively able to bind to the human colon carcinoma Caco-2 cell line and was phagocytosed by murine macrophage RAW 264.7, whereas the wild-type did not bind to epithelial cells and totally resisted internalization by macrophages.
Conclusions: Our results suggest that CPS/EPS production and fimbriae formation are negatively correlated and play key roles in the survival, attachment, and colonization of B. longum 105-A in the gut.
Keywords: Bifidobacterium longum 105-A; Caco-2 cell line; Capsular polysaccharides; Cell adhesion; Phagocytosis; RAW 264.7.
Figures
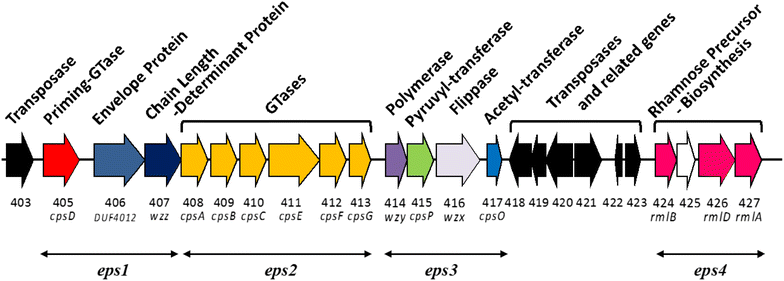

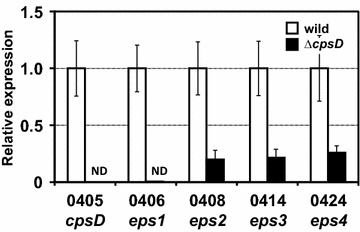
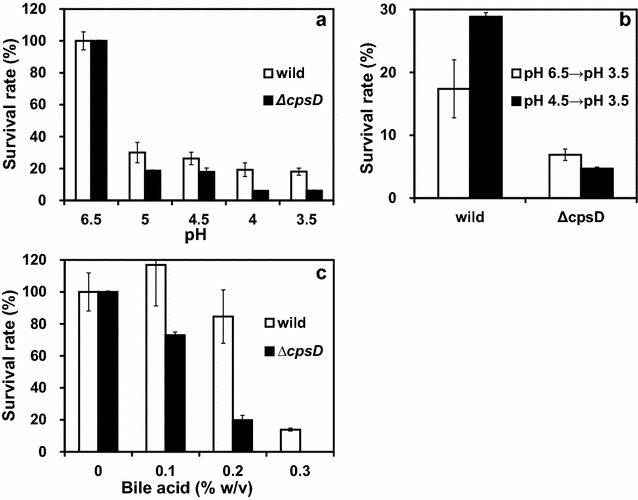
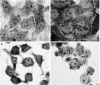
Similar articles
-
Sugar source modulates exopolysaccharide biosynthesis in Bifidobacterium longum subsp. longum CRC 002.Microbiology (Reading). 2010 Mar;156(Pt 3):653-664. doi: 10.1099/mic.0.033720-0. Epub 2009 Oct 22. Microbiology (Reading). 2010. PMID: 19850611
-
Impact of 4 Lactobacillus plantarum capsular polysaccharide clusters on surface glycan composition and host cell signaling.Microb Cell Fact. 2012 Nov 21;11:149. doi: 10.1186/1475-2859-11-149. Microb Cell Fact. 2012. PMID: 23170998 Free PMC article.
-
Streptococcus agalactiae capsule polymer length and attachment is determined by the proteins CpsABCD.J Biol Chem. 2015 Apr 10;290(15):9521-32. doi: 10.1074/jbc.M114.631499. Epub 2015 Feb 9. J Biol Chem. 2015. PMID: 25666613 Free PMC article.
-
Enhanced Acid Tolerance in Bifidobacterium longum by Adaptive Evolution: Comparison of the Genes between the Acid-Resistant Variant and Wild-Type Strain.J Microbiol Biotechnol. 2016 Mar;26(3):452-60. doi: 10.4014/jmb.1508.08030. J Microbiol Biotechnol. 2016. PMID: 26608165
-
Diversity and Function of Phage Encoded Depolymerases.Front Microbiol. 2020 Jan 10;10:2949. doi: 10.3389/fmicb.2019.02949. eCollection 2019. Front Microbiol. 2020. PMID: 31998258 Free PMC article. Review.
Cited by
-
Taxonomic and phenotypic analysis of bifidobacteria isolated from IBD patients as potential probiotic strains.BMC Microbiol. 2024 Jun 29;24(1):233. doi: 10.1186/s12866-024-03368-4. BMC Microbiol. 2024. PMID: 38951788 Free PMC article.
-
The distinction of CPR bacteria from other bacteria based on protein family content.Nat Commun. 2019 Sep 13;10(1):4173. doi: 10.1038/s41467-019-12171-z. Nat Commun. 2019. PMID: 31519891 Free PMC article.
-
Bacterial capsules: Occurrence, mechanism, and function.NPJ Biofilms Microbiomes. 2024 Mar 13;10(1):21. doi: 10.1038/s41522-024-00497-6. NPJ Biofilms Microbiomes. 2024. PMID: 38480745 Free PMC article. Review.
-
A programmable encapsulation system improves delivery of therapeutic bacteria in mice.Nat Biotechnol. 2022 Aug;40(8):1259-1269. doi: 10.1038/s41587-022-01244-y. Epub 2022 Mar 17. Nat Biotechnol. 2022. PMID: 35301496 Free PMC article.
-
Intracellular glycogen accumulation by human gut commensals as a niche adaptation trait.Gut Microbes. 2023 Jan-Dec;15(1):2235067. doi: 10.1080/19490976.2023.2235067. Gut Microbes. 2023. PMID: 37526383 Free PMC article. Review.
References
-
- Scholtens PAMJ, Oozeer R, Martin R, Amor K Ben, Knol J. The early settlers: Intestinal microbiology in early life. Annu Rev Food Sci Technol. 2012;3:425–47. http://www.annualreviews.org/doi/abs/10.1146/annurev-food-022811-101120. - DOI - PubMed
LinkOut - more resources
Full Text Sources
Other Literature Sources
Miscellaneous