Astrocytes locally translate transcripts in their peripheral processes
- PMID: 28439016
- PMCID: PMC5441704
- DOI: 10.1073/pnas.1617782114
Astrocytes locally translate transcripts in their peripheral processes
Abstract
Local translation in neuronal processes is key to the alteration of synaptic strength necessary for long-term potentiation, learning, and memory. Here, we present evidence that regulated de novo protein synthesis occurs within distal, perisynaptic astrocyte processes. Astrocyte ribosomal proteins are found adjacent to synapses in vivo, and immunofluorescent detection of peptide elongation in acute slices demonstrates robust translation in distal processes. We have also developed a biochemical approach to define candidate transcripts that are locally translated in astrocyte processes. Computational analyses indicate that astrocyte-localized translation is both sequence-dependent and enriched for particular biological functions, such as fatty acid synthesis, and for pathways consistent with known roles for astrocyte processes, such as GABA and glutamate metabolism. These transcripts also include glial regulators of synaptic refinement, such as Sparc Finally, the transcripts contain a disproportionate amount of a binding motif for the quaking RNA binding protein, a sequence we show can significantly regulate mRNA localization and translation in the astrocytes. Overall, our observations raise the possibility that local production of astrocyte proteins may support microscale alterations of adjacent synapses.
Keywords: RNA-sequencing; TRAP; astrocyte; local translation; synapse.
Conflict of interest statement
Conflict of interest statement: J.D.D. has received royalties for the translating ribosome affinity purification (TRAP) method.
Figures
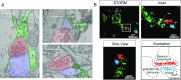
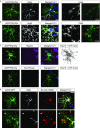
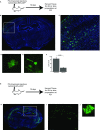
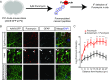
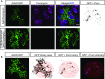
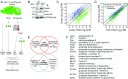
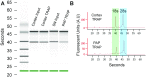
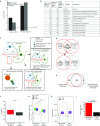
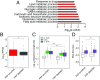
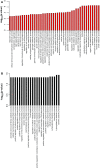
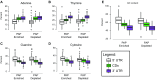
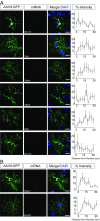
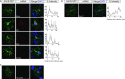
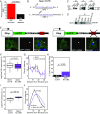
Similar articles
-
Pyridazine-derivatives Enhance Structural and Functional Plasticity of Tripartite Synapse Via Activation of Local Translation in Astrocytic Processes.Neuroscience. 2018 Sep 15;388:224-238. doi: 10.1016/j.neuroscience.2018.07.028. Epub 2018 Jul 27. Neuroscience. 2018. PMID: 30056115 Free PMC article.
-
Local translation in perisynaptic and perivascular astrocytic processes - a means to ensure astrocyte molecular and functional polarity?J Cell Sci. 2021 Jan 22;134(2):jcs251629. doi: 10.1242/jcs.251629. J Cell Sci. 2021. PMID: 33483366 Review.
-
Neuron-wide RNA transport combines with netrin-mediated local translation to spatially regulate the synaptic proteome.Elife. 2015 Jan 8;4:e04158. doi: 10.7554/eLife.04158. Elife. 2015. PMID: 25569157 Free PMC article.
-
Alterations in microglial phenotype and hippocampal neuronal function in transgenic mice with astrocyte-targeted production of interleukin-10.Brain Behav Immun. 2015 Mar;45:80-97. doi: 10.1016/j.bbi.2014.10.015. Epub 2014 Oct 31. Brain Behav Immun. 2015. PMID: 25449577
-
Cellular mechanisms of brain energy metabolism and their relevance to functional brain imaging.Philos Trans R Soc Lond B Biol Sci. 1999 Jul 29;354(1387):1155-63. doi: 10.1098/rstb.1999.0471. Philos Trans R Soc Lond B Biol Sci. 1999. PMID: 10466143 Free PMC article. Review.
Cited by
-
Cell-Type-Specific Profiling of Alternative Translation Identifies Regulated Protein Isoform Variation in the Mouse Brain.Cell Rep. 2019 Jan 15;26(3):594-607.e7. doi: 10.1016/j.celrep.2018.12.077. Cell Rep. 2019. PMID: 30650354 Free PMC article.
-
Learning and memory formation in zebrafish: Protein dynamics and molecular tools.Front Cell Dev Biol. 2023 Mar 9;11:1120984. doi: 10.3389/fcell.2023.1120984. eCollection 2023. Front Cell Dev Biol. 2023. PMID: 36968211 Free PMC article. Review.
-
Cooperative and competitive regulation of the astrocytic transcriptome by neurons and endothelial cells: Impact on astrocyte maturation.J Neurochem. 2023 Oct;167(1):52-75. doi: 10.1111/jnc.15908. Epub 2023 Jul 31. J Neurochem. 2023. PMID: 37525469 Free PMC article.
-
The US11 Gene of Herpes Simplex Virus 1 Promotes Neuroinvasion and Periocular Replication following Corneal Infection.J Virol. 2019 Apr 17;93(9):e02246-18. doi: 10.1128/JVI.02246-18. Print 2019 May 1. J Virol. 2019. PMID: 30760571 Free PMC article.
-
Calcium Signals in Astrocyte Microdomains, a Decade of Great Advances.Front Cell Neurosci. 2021 Jun 7;15:673433. doi: 10.3389/fncel.2021.673433. eCollection 2021. Front Cell Neurosci. 2021. PMID: 34163329 Free PMC article. Review.
References
-
- Araque A, Parpura V, Sanzgiri RP, Haydon PG. Tripartite synapses: Glia, the unacknowledged partner. Trends Neurosci. 1999;22:208–215. - PubMed
-
- Ullian EM, Sapperstein SK, Christopherson KS, Barres BA. Control of synapse number by glia. Science. 2001;291:657–661. - PubMed
-
- Mauch DH, et al. CNS synaptogenesis promoted by glia-derived cholesterol. Science. 2001;294:1354–1357. - PubMed
-
- Pfrieger FW, Barres BA. Synaptic efficacy enhanced by glial cells in vitro. Science. 1997;277:1684–1687. - PubMed
-
- Jourdain P, et al. Glutamate exocytosis from astrocytes controls synaptic strength. Nat Neurosci. 2007;10:331–339. - PubMed
Publication types
MeSH terms
Substances
Grants and funding
LinkOut - more resources
Full Text Sources
Other Literature Sources
Medical
Molecular Biology Databases
Miscellaneous