Quantitative Multiplex Immunohistochemistry Reveals Myeloid-Inflamed Tumor-Immune Complexity Associated with Poor Prognosis
- PMID: 28380359
- PMCID: PMC5564306
- DOI: 10.1016/j.celrep.2017.03.037
Quantitative Multiplex Immunohistochemistry Reveals Myeloid-Inflamed Tumor-Immune Complexity Associated with Poor Prognosis
Abstract
Here, we describe a multiplexed immunohistochemical platform with computational image processing workflows, including image cytometry, enabling simultaneous evaluation of 12 biomarkers in one formalin-fixed paraffin-embedded tissue section. To validate this platform, we used tissue microarrays containing 38 archival head and neck squamous cell carcinomas and revealed differential immune profiles based on lymphoid and myeloid cell densities, correlating with human papilloma virus status and prognosis. Based on these results, we investigated 24 pancreatic ductal adenocarcinomas from patients who received neoadjuvant GVAX vaccination and revealed that response to therapy correlated with degree of mono-myelocytic cell density and percentages of CD8+ T cells expressing T cell exhaustion markers. These data highlight the utility of in situ immune monitoring for patient stratification and provide digital image processing pipelines to the community for examining immune complexity in precious tissue sections, where phenotype and tissue architecture are preserved to improve biomarker discovery and assessment.
Keywords: cancer immunology; digital pathology; head and neck cancer; image cytometry; immunohistochemistry; multiplex; pancreatic cancer; tissue biomarker.
Copyright © 2017 The Author(s). Published by Elsevier Inc. All rights reserved.
Figures
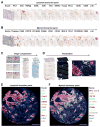
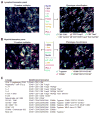
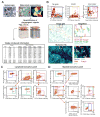
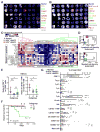
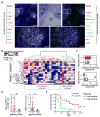
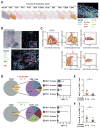
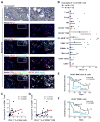
Similar articles
-
Robust Cell Detection and Segmentation for Image Cytometry Reveal Th17 Cell Heterogeneity.Cytometry A. 2019 Apr;95(4):389-398. doi: 10.1002/cyto.a.23726. Epub 2019 Feb 4. Cytometry A. 2019. PMID: 30714674 Free PMC article.
-
High-dimensional multiplexed immunohistochemical characterization of immune contexture in human cancers.Methods Enzymol. 2020;635:1-20. doi: 10.1016/bs.mie.2019.05.039. Epub 2019 Jul 2. Methods Enzymol. 2020. PMID: 32122539 Free PMC article.
-
Development of a programmed cell death ligand-1 immunohistochemical assay validated for analysis of non-small cell lung cancer and head and neck squamous cell carcinoma.Diagn Pathol. 2016 Oct 8;11(1):95. doi: 10.1186/s13000-016-0545-8. Diagn Pathol. 2016. PMID: 27717372 Free PMC article. Clinical Trial.
-
TILs in Head and Neck Cancer: Ready for Clinical Implementation and Why (Not)?Head Neck Pathol. 2017 Sep;11(3):354-363. doi: 10.1007/s12105-016-0776-8. Epub 2016 Dec 28. Head Neck Pathol. 2017. PMID: 28032290 Free PMC article. Review.
-
Advances in quantitative immunohistochemistry and their contribution to breast cancer.Expert Rev Mol Diagn. 2020 May;20(5):509-522. doi: 10.1080/14737159.2020.1743178. Epub 2020 Mar 30. Expert Rev Mol Diagn. 2020. PMID: 32178550 Review.
Cited by
-
Innate and adaptive immune-directed tumour microenvironment in pancreatic ductal adenocarcinoma.Front Immunol. 2024 Feb 7;15:1323198. doi: 10.3389/fimmu.2024.1323198. eCollection 2024. Front Immunol. 2024. PMID: 38384463 Free PMC article. Review.
-
Comprehensive Analysis of Myeloid Signature Genes in Head and Neck Squamous Cell Carcinoma to Predict the Prognosis and Immune Infiltration.Front Immunol. 2021 Apr 29;12:659184. doi: 10.3389/fimmu.2021.659184. eCollection 2021. Front Immunol. 2021. PMID: 33995379 Free PMC article.
-
Computational multiplex panel reduction to maximize information retention in breast cancer tissue microarrays.PLoS Comput Biol. 2022 Sep 30;18(9):e1010505. doi: 10.1371/journal.pcbi.1010505. eCollection 2022 Sep. PLoS Comput Biol. 2022. PMID: 36178966 Free PMC article.
-
Characterization of weaning-induced breast involution in women: implications for young women's breast cancer.NPJ Breast Cancer. 2020 Oct 16;6:55. doi: 10.1038/s41523-020-00196-3. eCollection 2020. NPJ Breast Cancer. 2020. PMID: 33083533 Free PMC article.
-
Single-cell morphodynamical trajectories enable prediction of gene expression accompanying cell state change.bioRxiv [Preprint]. 2024 Jun 25:2024.01.18.576248. doi: 10.1101/2024.01.18.576248. bioRxiv. 2024. PMID: 38293173 Free PMC article. Preprint.
References
-
- Fridman WH, Pages F, Sautes-Fridman C, Galon J. The immune contexture in human tumours: impact on clinical outcome. Nat Rev Cancer. 2012;12:298–306. - PubMed
-
- Galon J, Costes A, Sanchez-Cabo F, Kirilovsky A, Mlecnik B, Lagorce-Pages C, Tosolini M, Camus M, Berger A, Wind P, et al. Type, density, and location of immune cells within human colorectal tumors predict clinical outcome. Science. 2006;313:1960–1964. - PubMed
Publication types
MeSH terms
Substances
Grants and funding
LinkOut - more resources
Full Text Sources
Other Literature Sources
Medical
Research Materials