Transaldolase inhibition impairs mitochondrial respiration and induces a starvation-like longevity response in Caenorhabditis elegans
- PMID: 28355222
- PMCID: PMC5389855
- DOI: 10.1371/journal.pgen.1006695
Transaldolase inhibition impairs mitochondrial respiration and induces a starvation-like longevity response in Caenorhabditis elegans
Abstract
Mitochondrial dysfunction can increase oxidative stress and extend lifespan in Caenorhabditis elegans. Homeostatic mechanisms exist to cope with disruptions to mitochondrial function that promote cellular health and organismal longevity. Previously, we determined that decreased expression of the cytosolic pentose phosphate pathway (PPP) enzyme transaldolase activates the mitochondrial unfolded protein response (UPRmt) and extends lifespan. Here we report that transaldolase (tald-1) deficiency impairs mitochondrial function in vivo, as evidenced by altered mitochondrial morphology, decreased respiration, and increased cellular H2O2 levels. Lifespan extension from knockdown of tald-1 is associated with an oxidative stress response involving p38 and c-Jun N-terminal kinase (JNK) MAPKs and a starvation-like response regulated by the transcription factor EB (TFEB) homolog HLH-30. The latter response promotes autophagy and increases expression of the flavin-containing monooxygenase 2 (fmo-2). We conclude that cytosolic redox established through the PPP is a key regulator of mitochondrial function and defines a new mechanism for mitochondrial regulation of longevity.
Conflict of interest statement
The authors have declared that no competing interests exist.
Figures
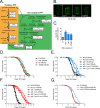
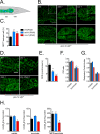
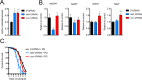
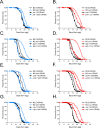
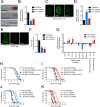
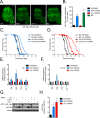
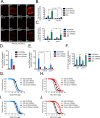
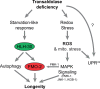
Similar articles
-
Mitochondrial translation and dynamics synergistically extend lifespan in C. elegans through HLH-30.J Cell Biol. 2020 Jun 1;219(6):e201907067. doi: 10.1083/jcb.201907067. J Cell Biol. 2020. PMID: 32259199 Free PMC article.
-
Radix Tetrastigma flavonoid ameliorates inflammation and prolongs the lifespan of Caenorhabditis elegans through JNK, p38 and Nrf2 pathways.Free Radic Res. 2019 May;53(5):562-573. doi: 10.1080/10715762.2019.1613534. Epub 2019 May 16. Free Radic Res. 2019. PMID: 31039619
-
Mitochondrial dysfunction, aging, and the mitochondrial unfolded protein response in Caenorhabditis elegans.Genetics. 2022 Nov 30;222(4):iyac160. doi: 10.1093/genetics/iyac160. Genetics. 2022. PMID: 36342845 Free PMC article. Review.
-
Nonselective autophagy reduces mitochondrial content during starvation in Caenorhabditis elegans.Am J Physiol Cell Physiol. 2018 Dec 1;315(6):C781-C792. doi: 10.1152/ajpcell.00109.2018. Epub 2018 Aug 22. Am J Physiol Cell Physiol. 2018. PMID: 30133321 Free PMC article.
-
The mitochondrial unfolded protein response and increased longevity: cause, consequence, or correlation?Exp Gerontol. 2014 Aug;56:142-6. doi: 10.1016/j.exger.2014.02.002. Epub 2014 Feb 8. Exp Gerontol. 2014. PMID: 24518875 Free PMC article. Review.
Cited by
-
C. elegans orphan nuclear receptor NHR-42 represses innate immunity and promotes lipid loss downstream of HLH-30/TFEB.Front Immunol. 2023 Feb 13;14:1094145. doi: 10.3389/fimmu.2023.1094145. eCollection 2023. Front Immunol. 2023. PMID: 36860863 Free PMC article.
-
Emerging topics in C. elegans aging research: Transcriptional regulation, stress response and epigenetics.Mech Ageing Dev. 2019 Jan;177:4-21. doi: 10.1016/j.mad.2018.08.001. Epub 2018 Aug 19. Mech Ageing Dev. 2019. PMID: 30134144 Free PMC article. Review.
-
Mitochondrial quality control in acute ischemic stroke.J Cereb Blood Flow Metab. 2021 Dec;41(12):3157-3170. doi: 10.1177/0271678X211046992. Epub 2021 Sep 22. J Cereb Blood Flow Metab. 2021. PMID: 34551609 Free PMC article. Review.
-
Shaping longevity early in life: developmental ROS and H3K4me3 set the clock.Cell Cycle. 2021 Nov;20(22):2337-2347. doi: 10.1080/15384101.2021.1986317. Epub 2021 Oct 17. Cell Cycle. 2021. PMID: 34657571 Free PMC article.
-
Comprehensive analysis of gene expression profiles associated with proliferative diabetic retinopathy.Exp Ther Med. 2018 Oct;16(4):3539-3545. doi: 10.3892/etm.2018.6635. Epub 2018 Aug 21. Exp Ther Med. 2018. PMID: 30250524 Free PMC article.
References
-
- Harman D. The biologic clock: the mitochondria? J Am Geriatr Soc. 1972;20(4):145–7. - PubMed
Publication types
MeSH terms
Substances
Grants and funding
LinkOut - more resources
Full Text Sources
Other Literature Sources
Research Materials
Miscellaneous