Cell membrane-derived nanomaterials for biomedical applications
- PMID: 28292726
- PMCID: PMC5417338
- DOI: 10.1016/j.biomaterials.2017.02.041
Cell membrane-derived nanomaterials for biomedical applications
Abstract
The continued evolution of biomedical nanotechnology has enabled clinicians to better detect, prevent, manage, and treat human disease. In order to further push the limits of nanoparticle performance and functionality, there has recently been a paradigm shift towards biomimetic design strategies. By taking inspiration from nature, the goal is to create next-generation nanoparticle platforms that can more effectively navigate and interact with the incredibly complex biological systems that exist within the body. Of great interest are cellular membranes, which play essential roles in biointerfacing, self-identification, signal transduction, and compartmentalization. In this review, we explore the major ways in which researchers have directly leveraged cell membrane-derived biomaterials for the fabrication of novel nanotherapeutics and nanodiagnostics. Such emerging technologies have the potential to significantly advance the field of nanomedicine, helping to improve upon traditional modalities while also enabling novel applications.
Keywords: Biomimetic nanoparticle; Cell membrane; Detoxification; Drug delivery; Imaging; Immunotherapy.
Copyright © 2017 Elsevier Ltd. All rights reserved.
Figures
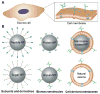
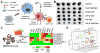
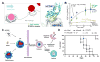
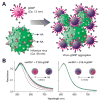
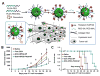
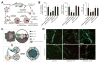
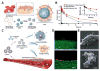
Similar articles
-
Rationally Programming Nanomaterials with DNA for Biomedical Applications.Adv Sci (Weinh). 2021 Feb 24;8(8):2003775. doi: 10.1002/advs.202003775. eCollection 2021 Apr. Adv Sci (Weinh). 2021. PMID: 33898180 Free PMC article. Review.
-
The applications of conductive nanomaterials in the biomedical field.J Biomed Mater Res A. 2016 Jan;104(1):322-39. doi: 10.1002/jbm.a.35537. Epub 2015 Aug 8. J Biomed Mater Res A. 2016. PMID: 26179845 Review.
-
Recent Advances of Membrane-Cloaked Nanoplatforms for Biomedical Applications.Bioconjug Chem. 2018 Apr 18;29(4):838-851. doi: 10.1021/acs.bioconjchem.8b00103. Epub 2018 Mar 20. Bioconjug Chem. 2018. PMID: 29509403 Review.
-
Cell Membrane Coating Nanotechnology.Adv Mater. 2018 Jun;30(23):e1706759. doi: 10.1002/adma.201706759. Epub 2018 Mar 27. Adv Mater. 2018. PMID: 29582476 Free PMC article. Review.
-
Biomedical applications of 2D monoelemental materials formed by group VA and VIA: a concise review.J Nanobiotechnology. 2021 Apr 1;19(1):96. doi: 10.1186/s12951-021-00825-4. J Nanobiotechnology. 2021. PMID: 33794908 Free PMC article. Review.
Cited by
-
Interactions Between Tumor Biology and Targeted Nanoplatforms for Imaging Applications.Adv Funct Mater. 2020 May 11;30(19):1910402. doi: 10.1002/adfm.201910402. Epub 2020 Mar 3. Adv Funct Mater. 2020. PMID: 34093104 Free PMC article.
-
Neddylation suppression by a macrophage membrane-coated nanoparticle promotes dual immunomodulatory repair of diabetic wounds.Bioact Mater. 2024 Jan 6;34:366-380. doi: 10.1016/j.bioactmat.2023.12.025. eCollection 2024 Apr. Bioact Mater. 2024. PMID: 38269308 Free PMC article.
-
Cell Membrane Coating Technology: A Promising Strategy for Biomedical Applications.Nanomicro Lett. 2019 Nov 16;11(1):100. doi: 10.1007/s40820-019-0330-9. Nanomicro Lett. 2019. PMID: 34138027 Free PMC article. Review.
-
Platelet bio-nanobubbles as microvascular recanalization nanoformulation for acute ischemic stroke lesion theranostics.Theranostics. 2018 Sep 9;8(18):4870-4883. doi: 10.7150/thno.27466. eCollection 2018. Theranostics. 2018. PMID: 30429874 Free PMC article.
-
Engineering biological interactions on the nanoscale.Curr Opin Biotechnol. 2019 Aug;58:1-8. doi: 10.1016/j.copbio.2018.10.005. Epub 2018 Nov 1. Curr Opin Biotechnol. 2019. PMID: 30390535 Free PMC article. Review.
References
Publication types
MeSH terms
Grants and funding
LinkOut - more resources
Full Text Sources
Other Literature Sources