C-edge loops of arrestin function as a membrane anchor
- PMID: 28220785
- PMCID: PMC5321764
- DOI: 10.1038/ncomms14258
C-edge loops of arrestin function as a membrane anchor
Abstract
G-protein-coupled receptors are membrane proteins that are regulated by a small family of arrestin proteins. During formation of the arrestin-receptor complex, arrestin first interacts with the phosphorylated receptor C terminus in a pre-complex, which activates arrestin for tight receptor binding. Currently, little is known about the structure of the pre-complex and its transition to a high-affinity complex. Here we present molecular dynamics simulations and site-directed fluorescence experiments on arrestin-1 interactions with rhodopsin, showing that loops within the C-edge of arrestin function as a membrane anchor. Activation of arrestin by receptor-attached phosphates is necessary for C-edge engagement of the membrane, and we show that these interactions are distinct in the pre-complex and high-affinity complex in regard to their conformation and orientation. Our results expand current knowledge of C-edge structure and further illuminate the conformational transitions that occur in arrestin along the pathway to tight receptor binding.
Conflict of interest statement
The authors declare no competing financial interests.
Figures
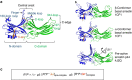
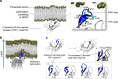
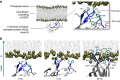
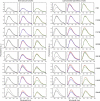
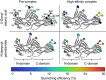
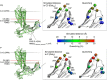
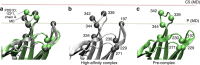
Similar articles
-
Arrestin-1 engineering facilitates complex stabilization with native rhodopsin.Sci Rep. 2019 Jan 24;9(1):439. doi: 10.1038/s41598-018-36881-4. Sci Rep. 2019. PMID: 30679635 Free PMC article.
-
Conformational changes in the phosphorylated C-terminal domain of rhodopsin during rhodopsin arrestin interactions.J Biol Chem. 2004 Dec 3;279(49):51203-7. doi: 10.1074/jbc.M407341200. Epub 2004 Sep 6. J Biol Chem. 2004. PMID: 15351781
-
The differential engagement of arrestin surface charges by the various functional forms of the receptor.J Biol Chem. 2006 Feb 10;281(6):3458-62. doi: 10.1074/jbc.M512148200. Epub 2005 Dec 8. J Biol Chem. 2006. PMID: 16339758 Free PMC article.
-
Structural Basis of Arrestin Selectivity for Active Phosphorylated G Protein-Coupled Receptors.Int J Mol Sci. 2021 Nov 19;22(22):12481. doi: 10.3390/ijms222212481. Int J Mol Sci. 2021. PMID: 34830362 Free PMC article. Review.
-
A structural snapshot of the rhodopsin-arrestin complex.FEBS J. 2016 Mar;283(5):816-21. doi: 10.1111/febs.13561. Epub 2015 Nov 7. FEBS J. 2016. PMID: 26467309 Free PMC article. Review.
Cited by
-
Membrane phosphoinositides regulate GPCR-β-arrestin complex assembly and dynamics.Cell. 2022 Nov 23;185(24):4560-4573.e19. doi: 10.1016/j.cell.2022.10.018. Epub 2022 Nov 10. Cell. 2022. PMID: 36368322 Free PMC article.
-
Structure of the neurotensin receptor 1 in complex with β-arrestin 1.Nature. 2020 Mar;579(7798):303-308. doi: 10.1038/s41586-020-1953-1. Epub 2020 Jan 16. Nature. 2020. PMID: 31945771 Free PMC article.
-
Lipid nanoparticle technologies for the study of G protein-coupled receptors in lipid environments.Biophys Rev. 2020 Nov 19;12(6):1287-302. doi: 10.1007/s12551-020-00775-5. Online ahead of print. Biophys Rev. 2020. PMID: 33215301 Free PMC article. Review.
-
Mechanism of β-arrestin recruitment by the μ-opioid G protein-coupled receptor.Proc Natl Acad Sci U S A. 2020 Jul 14;117(28):16346-16355. doi: 10.1073/pnas.1918264117. Epub 2020 Jun 29. Proc Natl Acad Sci U S A. 2020. PMID: 32601232 Free PMC article.
-
G protein-coupled receptor interactions with arrestins and GPCR kinases: The unresolved issue of signal bias.J Biol Chem. 2022 Sep;298(9):102279. doi: 10.1016/j.jbc.2022.102279. Epub 2022 Jul 19. J Biol Chem. 2022. PMID: 35863432 Free PMC article. Review.
References
Publication types
MeSH terms
Substances
LinkOut - more resources
Full Text Sources
Other Literature Sources