Liquid behavior of cross-linked actin bundles
- PMID: 28202730
- PMCID: PMC5338483
- DOI: 10.1073/pnas.1616133114
Liquid behavior of cross-linked actin bundles
Abstract
The actin cytoskeleton is a critical regulator of cytoplasmic architecture and mechanics, essential in a myriad of physiological processes. Here we demonstrate a liquid phase of actin filaments in the presence of the physiological cross-linker, filamin. Filamin condenses short actin filaments into spindle-shaped droplets, or tactoids, with shape dynamics consistent with a continuum model of anisotropic liquids. We find that cross-linker density controls the droplet shape and deformation timescales, consistent with a variable interfacial tension and viscosity. Near the liquid-solid transition, cross-linked actin bundles show behaviors reminiscent of fluid threads, including capillary instabilities and contraction. These data reveal a liquid droplet phase of actin, demixed from the surrounding solution and dominated by interfacial tension. These results suggest a mechanism to control organization, morphology, and dynamics of the actin cytoskeleton.
Keywords: actin; cytoskeleton; liquid crystal; phase separation.
Conflict of interest statement
The authors declare no conflict of interest.
Figures
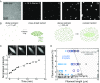
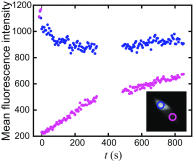
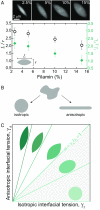

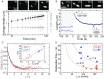
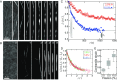
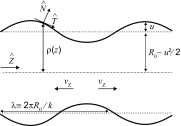
Similar articles
-
Tuning shape and internal structure of protein droplets via biopolymer filaments.Soft Matter. 2020 Jun 24;16(24):5659-5668. doi: 10.1039/c9sm02462j. Soft Matter. 2020. PMID: 32519715
-
Discontinuous unbinding transitions of filament bundles.Phys Rev Lett. 2005 Jul 15;95(3):038102. doi: 10.1103/PhysRevLett.95.038102. Epub 2005 Jul 14. Phys Rev Lett. 2005. PMID: 16090774
-
Filament rigidity and connectivity tune the deformation modes of active biopolymer networks.Proc Natl Acad Sci U S A. 2017 Nov 21;114(47):E10037-E10045. doi: 10.1073/pnas.1708625114. Epub 2017 Nov 7. Proc Natl Acad Sci U S A. 2017. PMID: 29114058 Free PMC article.
-
ADP-Ribosylation and Cross-Linking of Actin by Bacterial Protein Toxins.Handb Exp Pharmacol. 2017;235:179-206. doi: 10.1007/164_2016_26. Handb Exp Pharmacol. 2017. PMID: 27316913 Review.
-
Advances in the mechanical modeling of filamentous actin and its cross-linked networks on multiple scales.Biomech Model Mechanobiol. 2014 Nov;13(6):1155-74. doi: 10.1007/s10237-014-0578-4. Epub 2014 Apr 4. Biomech Model Mechanobiol. 2014. PMID: 24700235 Review.
Cited by
-
A proposed role for the SARS-CoV-2 nucleocapsid protein in the formation and regulation of biomolecular condensates.FASEB J. 2020 Aug;34(8):9832-9842. doi: 10.1096/fj.202001351. Epub 2020 Jun 20. FASEB J. 2020. PMID: 32562316 Free PMC article.
-
Multiscale Modeling of Protein-RNA Condensation in and Out of Equilibrium.Methods Mol Biol. 2023;2563:117-133. doi: 10.1007/978-1-0716-2663-4_5. Methods Mol Biol. 2023. PMID: 36227470 Free PMC article.
-
Metabolic Compartmentalization at the Leading Edge of Metastatic Cancer Cells.Front Oncol. 2020 Nov 2;10:554272. doi: 10.3389/fonc.2020.554272. eCollection 2020. Front Oncol. 2020. PMID: 33224873 Free PMC article. Review.
-
Capillary forces generated by biomolecular condensates.Nature. 2022 Sep;609(7926):255-264. doi: 10.1038/s41586-022-05138-6. Epub 2022 Sep 7. Nature. 2022. PMID: 36071192 Review.
-
Anillin propels myosin-independent constriction of actin rings.Nat Commun. 2021 Jul 28;12(1):4595. doi: 10.1038/s41467-021-24474-1. Nat Commun. 2021. PMID: 34321459 Free PMC article.
References
-
- Alberts B, et al. Molecular Biology of the Cell. 6th Ed. Garland Science; New York: 2015. pp. 1–1342.
-
- Lecuit T, Lenne PF, Munro E. Force generation, transmission, and integration during cell and tissue morphogenesis. Annu Rev Cell Dev Biol. 2011;27(27):157–184. - PubMed
-
- Blanchoin L, Boujemaa-Paterski R, Sykes C, Plastino J. Actin dynamics, architecture, and mechanics in cell motility. Physiol Rev. 2014;94(1):235–263. - PubMed
-
- Prost J, Julicher F, Joanny JF. Active gel physics. Nat Phys. 2015;11(2):111–117.
Publication types
MeSH terms
Substances
LinkOut - more resources
Full Text Sources
Other Literature Sources