Action potentials and ion conductances in wild-type and CALHM1-knockout type II taste cells
- PMID: 28202574
- PMCID: PMC5411462
- DOI: 10.1152/jn.00835.2016
Action potentials and ion conductances in wild-type and CALHM1-knockout type II taste cells
Abstract
Taste bud type II cells fire action potentials in response to tastants, triggering nonvesicular ATP release to gustatory neurons via voltage-gated CALHM1-associated ion channels. Whereas CALHM1 regulates mouse cortical neuron excitability, its roles in regulating type II cell excitability are unknown. In this study, we compared membrane conductances and action potentials in single identified TRPM5-GFP-expressing circumvallate papillae type II cells acutely isolated from wild-type (WT) and Calhm1 knockout (KO) mice. The activation kinetics of large voltage-gated outward currents were accelerated in cells from Calhm1 KO mice, and their associated nonselective tail currents, previously shown to be highly correlated with ATP release, were completely absent in Calhm1 KO cells, suggesting that CALHM1 contributes to all of these currents. Calhm1 deletion did not significantly alter resting membrane potential or input resistance, the amplitudes and kinetics of Na+ currents either estimated from action potentials or recorded from steady-state voltage pulses, or action potential threshold, overshoot peak, afterhyperpolarization, and firing frequency. However, Calhm1 deletion reduced the half-widths of action potentials and accelerated the deactivation kinetics of transient outward currents, suggesting that the CALHM1-associated conductance becomes activated during the repolarization phase of action potentials.NEW & NOTEWORTHY CALHM1 is an essential ion channel component of the ATP neurotransmitter release mechanism in type II taste bud cells. Its contribution to type II cell resting membrane properties and excitability is unknown. Nonselective voltage-gated currents, previously associated with ATP release, were absent in cells lacking CALHM1. Calhm1 deletion was without effects on resting membrane properties or voltage-gated Na+ and K+ channels but contributed modestly to the kinetics of action potentials.
Keywords: ATP release channel; taste bud; voltage clamp; voltage-gated ion channel.
Copyright © 2017 the American Physiological Society.
Figures
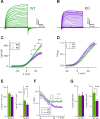
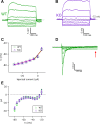
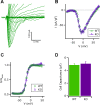
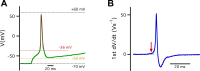
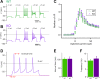
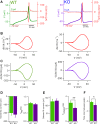
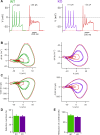
Similar articles
-
CALHM1 ion channel mediates purinergic neurotransmission of sweet, bitter and umami tastes.Nature. 2013 Mar 14;495(7440):223-6. doi: 10.1038/nature11906. Epub 2013 Mar 6. Nature. 2013. PMID: 23467090 Free PMC article.
-
How do taste cells lacking synapses mediate neurotransmission? CALHM1, a voltage-gated ATP channel.Bioessays. 2013 Dec;35(12):1111-8. doi: 10.1002/bies.201300077. Epub 2013 Sep 17. Bioessays. 2013. PMID: 24105910 Free PMC article. Review.
-
Characteristics of action potentials and their underlying outward currents in rat taste receptor cells.J Neurophysiol. 1996 Feb;75(2):820-31. doi: 10.1152/jn.1996.75.2.820. J Neurophysiol. 1996. PMID: 8714655
-
Amiloride-sensitive channels in type I fungiform taste cells in mouse.BMC Neurosci. 2008 Jan 2;9:1. doi: 10.1186/1471-2202-9-1. BMC Neurosci. 2008. PMID: 18171468 Free PMC article.
-
Calcium homeostasis modulator (CALHM) ion channels.Pflugers Arch. 2016 Mar;468(3):395-403. doi: 10.1007/s00424-015-1757-6. Epub 2015 Nov 25. Pflugers Arch. 2016. PMID: 26603282 Free PMC article. Review.
Cited by
-
The NH2 terminus regulates voltage-dependent gating of CALHM ion channels.Am J Physiol Cell Physiol. 2017 Aug 1;313(2):C173-C186. doi: 10.1152/ajpcell.00318.2016. Epub 2017 May 17. Am J Physiol Cell Physiol. 2017. PMID: 28515089 Free PMC article.
-
CALHM3 Is Essential for Rapid Ion Channel-Mediated Purinergic Neurotransmission of GPCR-Mediated Tastes.Neuron. 2018 May 2;98(3):547-561.e10. doi: 10.1016/j.neuron.2018.03.043. Epub 2018 Apr 19. Neuron. 2018. PMID: 29681531 Free PMC article.
-
Taste transduction and channel synapses in taste buds.Pflugers Arch. 2021 Jan;473(1):3-13. doi: 10.1007/s00424-020-02464-4. Epub 2020 Sep 16. Pflugers Arch. 2021. PMID: 32936320 Free PMC article. Review.
-
Systematic Identification of MCU Modulators by Orthogonal Interspecies Chemical Screening.Mol Cell. 2017 Aug 17;67(4):711-723.e7. doi: 10.1016/j.molcel.2017.07.019. Mol Cell. 2017. PMID: 28820965 Free PMC article.
-
ATP Release Channels.Int J Mol Sci. 2018 Mar 11;19(3):808. doi: 10.3390/ijms19030808. Int J Mol Sci. 2018. PMID: 29534490 Free PMC article. Review.
References
Publication types
MeSH terms
Substances
Grants and funding
LinkOut - more resources
Full Text Sources
Other Literature Sources
Molecular Biology Databases
Research Materials