Spatial and Temporal Analysis of Alphavirus Replication and Assembly in Mammalian and Mosquito Cells
- PMID: 28196962
- PMCID: PMC5312085
- DOI: 10.1128/mBio.02294-16
Spatial and Temporal Analysis of Alphavirus Replication and Assembly in Mammalian and Mosquito Cells
Abstract
Sindbis virus (SINV [genus Alphavirus, family Togaviridae]) is an enveloped, mosquito-borne virus. Alphaviruses cause cytolytic infections in mammalian cells while establishing noncytopathic, persistent infections in mosquito cells. Mosquito vector adaptation of alphaviruses is a major factor in the transmission of epidemic strains of alphaviruses. Though extensive studies have been performed on infected mammalian cells, the morphological and structural elements of alphavirus replication and assembly remain poorly understood in mosquito cells. Here we used high-resolution live-cell imaging coupled with single-particle tracking and electron microscopy analyses to delineate steps in the alphavirus life cycle in both the mammalian host cell and insect vector cells. Use of dually labeled SINV in conjunction with cellular stains enabled us to simultaneously determine the spatial and temporal differences of alphavirus replication complexes (RCs) in mammalian and insect cells. We found that the nonstructural viral proteins and viral RNA in RCs exhibit distinct spatial organization in mosquito cytopathic vacuoles compared to replication organelles from mammalian cells. We show that SINV exploits filopodial extensions for virus dissemination in both cell types. Additionally, we propose a novel mechanism for replication complex formation around glycoprotein-containing vesicles in mosquito cells that produced internally released particles that were seen budding from the vesicles by live imaging. Finally, by characterizing mosquito cell lines that were persistently infected with fluorescent virus, we show that the replication and assembly machinery are highly modified, and this allows continuous production of alphaviruses at reduced levels.IMPORTANCE Reemerging mosquito-borne alphaviruses cause serious human epidemics worldwide. Several structural and imaging studies have helped to define the life cycle of alphaviruses in mammalian cells, but the mode of virus replication and assembly in the invertebrate vector and mechanisms producing two disease outcomes in two types of cells are yet to be identified. Using transmission electron microscopy and live-cell imaging with dual fluorescent protein-tagged SINV, we show that while insect and mammalian cells display similarities in entry and exit, they present distinct spatial and temporal organizations in virus replication and assembly. By characterizing acutely and persistently infected cells, we provide new insights into alphavirus replication and assembly in two distinct hosts, resulting in high-titer virus production in mammalian cells and continuous virus production at reduced levels in mosquito cells-presumably a prerequisite for alphavirus maintenance in nature.
Copyright © 2017 Jose et al.
Figures
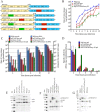
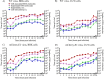
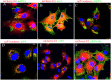
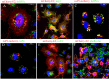
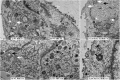
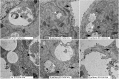
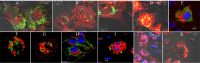
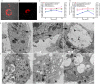
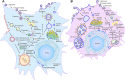
Similar articles
-
Alphavirus transducing system: tools for visualizing infection in mosquito vectors.J Vis Exp. 2010 Nov 24;(45):2363. doi: 10.3791/2363. J Vis Exp. 2010. PMID: 21178952 Free PMC article.
-
Effects of inducing or inhibiting apoptosis on Sindbis virus replication in mosquito cells.J Gen Virol. 2008 Nov;89(Pt 11):2651-2661. doi: 10.1099/vir.0.2008/005314-0. J Gen Virol. 2008. PMID: 18931060 Free PMC article.
-
Comparative Characterization of the Sindbis Virus Proteome from Mammalian and Invertebrate Hosts Identifies nsP2 as a Component of the Virion and Sorting Nexin 5 as a Significant Host Factor for Alphavirus Replication.J Virol. 2018 Jun 29;92(14):e00694-18. doi: 10.1128/JVI.00694-18. Print 2018 Jul 15. J Virol. 2018. PMID: 29743363 Free PMC article.
-
The Alphavirus Exit Pathway: What We Know and What We Wish We Knew.Viruses. 2018 Feb 22;10(2):89. doi: 10.3390/v10020089. Viruses. 2018. PMID: 29470397 Free PMC article. Review.
-
Persistent infection and suppression of host response by alphaviruses.Arch Virol Suppl. 2004;(18):139-47. doi: 10.1007/978-3-7091-0572-6_12. Arch Virol Suppl. 2004. PMID: 15119769 Review.
Cited by
-
PERK Is Critical for Alphavirus Nonstructural Protein Translation.Viruses. 2021 May 12;13(5):892. doi: 10.3390/v13050892. Viruses. 2021. PMID: 34065980 Free PMC article.
-
Role of the Guanine Nucleotide Exchange Factor GBF1 in the Replication of RNA Viruses.Viruses. 2020 Jun 24;12(6):682. doi: 10.3390/v12060682. Viruses. 2020. PMID: 32599855 Free PMC article. Review.
-
Genomic and In Vitro Phenotypic Comparisons of Epidemic and Non-Epidemic Getah Virus Strains.Viruses. 2022 Apr 30;14(5):942. doi: 10.3390/v14050942. Viruses. 2022. PMID: 35632684 Free PMC article.
-
Disentangling the Frames, the State of Research on the Alphavirus 6K and TF Proteins.Viruses. 2017 Aug 18;9(8):228. doi: 10.3390/v9080228. Viruses. 2017. PMID: 28820485 Free PMC article. Review.
-
Quantitative measurements of early alphaviral replication dynamics in single cells reveals the basis for superinfection exclusion.Cell Syst. 2021 Mar 17;12(3):210-219.e3. doi: 10.1016/j.cels.2020.12.005. Epub 2021 Jan 29. Cell Syst. 2021. PMID: 33515490 Free PMC article.
References
Publication types
MeSH terms
Substances
Grants and funding
LinkOut - more resources
Full Text Sources
Other Literature Sources