Molecular determinants of the N-terminal acetyltransferase Naa60 anchoring to the Golgi membrane
- PMID: 28196861
- PMCID: PMC5399128
- DOI: 10.1074/jbc.M116.770362
Molecular determinants of the N-terminal acetyltransferase Naa60 anchoring to the Golgi membrane
Abstract
Nα-Acetyltransferase 60 (Naa60 or NatF) was recently identified as an unconventional N-terminal acetyltransferase (NAT) because it localizes to organelles, in particular the Golgi apparatus, and has a preference for acetylating N termini of the transmembrane proteins. This knowledge challenged the prevailing view of N-terminal acetylation as a co-translational ribosome-associated process and suggested a new mechanistic functioning for the enzymes responsible for this increasingly recognized protein modification. Crystallography studies on Naa60 were unable to resolve the C-terminal tail of Naa60, which is responsible for the organellar localization. Here, we combined modeling, in vitro assays, and cellular localization studies to investigate the secondary structure and membrane interacting capacity of Naa60. The results show that Naa60 is a peripheral membrane protein. Two amphipathic helices within the Naa60 C terminus bind the membrane directly in a parallel position relative to the lipid bilayer via hydrophobic and electrostatic interactions. A peptide corresponding to the C terminus was unstructured in solution and only folded into an α-helical conformation in the presence of liposomes. Computational modeling and cellular mutational analysis revealed the hydrophobic face of two α-helices to be critical for membranous localization. Furthermore, we found a strong and specific binding preference of Naa60 toward membranes containing the phosphatidylinositol PI(4)P, thus possibly explaining the primary residency of Naa60 at the PI(4)P-rich Golgi. In conclusion, we have defined the mode of cytosolic Naa60 anchoring to the Golgi apparatus, most likely occurring post-translationally and specifically facilitating post-translational N-terminal acetylation of many transmembrane proteins.
Keywords: Golgi; N-terminal acetylation; NAT; Naa60; NatF; PI(4)P; acetylation; acetyltransferase; lipid binding protein; membrane enzyme.
© 2017 by The American Society for Biochemistry and Molecular Biology, Inc.
Conflict of interest statement
The authors declare that they have no conflicts of interest with the contents of this article
Figures
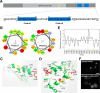
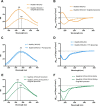
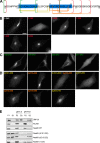
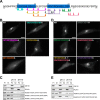
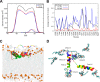
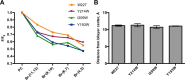
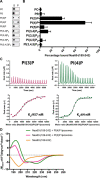
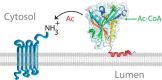
Similar articles
-
An organellar nα-acetyltransferase, naa60, acetylates cytosolic N termini of transmembrane proteins and maintains Golgi integrity.Cell Rep. 2015 Mar 3;10(8):1362-74. doi: 10.1016/j.celrep.2015.01.053. Epub 2015 Feb 26. Cell Rep. 2015. PMID: 25732826
-
Crystal Structure of the Golgi-Associated Human Nα-Acetyltransferase 60 Reveals the Molecular Determinants for Substrate-Specific Acetylation.Structure. 2016 Jul 6;24(7):1044-56. doi: 10.1016/j.str.2016.04.020. Epub 2016 Jun 16. Structure. 2016. PMID: 27320834 Free PMC article.
-
Structure and function of human Naa60 (NatF), a Golgi-localized bi-functional acetyltransferase.Sci Rep. 2016 Aug 23;6:31425. doi: 10.1038/srep31425. Sci Rep. 2016. PMID: 27550639 Free PMC article.
-
NAA60 (HAT4): the newly discovered bi-functional Golgi member of the acetyltransferase family.Clin Epigenetics. 2022 Dec 21;14(1):182. doi: 10.1186/s13148-022-01402-8. Clin Epigenetics. 2022. PMID: 36539894 Free PMC article. Review.
-
Co-translational, Post-translational, and Non-catalytic Roles of N-Terminal Acetyltransferases.Mol Cell. 2019 Mar 21;73(6):1097-1114. doi: 10.1016/j.molcel.2019.02.007. Epub 2019 Mar 13. Mol Cell. 2019. PMID: 30878283 Free PMC article. Review.
Cited by
-
Phospholipid Membrane Interactions of Model Ac-WL-X-LL-OH Peptides Investigated by Solid-State Nuclear Magnetic Resonance.Membranes (Basel). 2024 May 1;14(5):105. doi: 10.3390/membranes14050105. Membranes (Basel). 2024. PMID: 38786939 Free PMC article.
-
The function of histone acetylation in cervical cancer development.Biosci Rep. 2019 Apr 12;39(4):BSR20190527. doi: 10.1042/BSR20190527. Print 2019 Apr 30. Biosci Rep. 2019. PMID: 30886064 Free PMC article. Review.
-
Dynamics-function relationship in the catalytic domains of N-terminal acetyltransferases.Comput Struct Biotechnol J. 2020 Mar 3;18:532-547. doi: 10.1016/j.csbj.2020.02.017. eCollection 2020. Comput Struct Biotechnol J. 2020. PMID: 32206212 Free PMC article.
-
Specificity of Loxosceles α clade phospholipase D enzymes for choline-containing lipids: Role of a conserved aromatic cage.PLoS Comput Biol. 2022 Feb 18;18(2):e1009871. doi: 10.1371/journal.pcbi.1009871. eCollection 2022 Feb. PLoS Comput Biol. 2022. PMID: 35180220 Free PMC article.
-
Extended N-Terminal Acetyltransferase Naa50 in Filamentous Fungi Adds to Naa50 Diversity.Int J Mol Sci. 2022 Sep 16;23(18):10805. doi: 10.3390/ijms231810805. Int J Mol Sci. 2022. PMID: 36142717 Free PMC article.
References
-
- Aksnes H., Drazic A., Marie M., and Arnesen T. (2016) First things first: vital protein marks by N-terminal acetyltransferases. Trends Biochem. Sci. 41, 746–760 - PubMed
-
- Drazic A., Myklebust L. M., Ree R., and Arnesen T. (2016) The world of protein acetylation. Biochim. Biophys. Acta 1864, 1372–1401 - PubMed
-
- Polevoda B., Brown S., Cardillo T. S., Rigby S., and Sherman F. (2008) Yeast N(α)-terminal acetyltransferases are associated with ribosomes. J. Cell Biochem. 103, 492–508 - PubMed
MeSH terms
Substances
Associated data
- Actions
LinkOut - more resources
Full Text Sources
Other Literature Sources
Molecular Biology Databases
Research Materials
Miscellaneous