Serine ADP-Ribosylation Depends on HPF1
- PMID: 28190768
- PMCID: PMC5344681
- DOI: 10.1016/j.molcel.2017.01.003
Serine ADP-Ribosylation Depends on HPF1
Abstract
ADP-ribosylation (ADPr) regulates important patho-physiological processes through its attachment to different amino acids in proteins. Recently, by precision mapping on all possible amino acid residues, we identified histone serine ADPr marks in the DNA damage response. However, the biochemical basis underlying this serine modification remained unknown. Here we report that serine ADPr is strictly dependent on histone PARylation factor 1 (HPF1), a recently identified regulator of PARP-1. Quantitative proteomics revealed that serine ADPr does not occur in cells lacking HPF1. Moreover, adding HPF1 to in vitro PARP-1/PARP-2 reactions is necessary and sufficient for serine-specific ADPr of histones and PARP-1 itself. Three endogenous serine ADPr sites are located on the PARP-1 automodification domain. Further identification of serine ADPr on HMG proteins and hundreds of other targets indicates that serine ADPr is a widespread modification. We propose that O-linked protein ADPr is the key signal in PARP-1/PARP-2-dependent processes that govern genome stability.
Keywords: ADP-ribosylation; DNA damage; HPF1; PARP-1; PARP-2; genome stability; histones; proteomics; serine ADP-ribosylation.
Copyright © 2017 The Authors. Published by Elsevier Inc. All rights reserved.
Figures
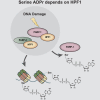
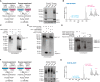
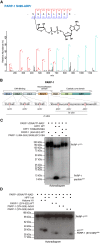
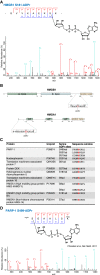
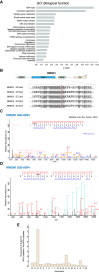
Comment in
-
ADP-Ribosylation Goes Normal: Serine as the Major Site of the Modification.Cell Chem Biol. 2017 Apr 20;24(4):431-432. doi: 10.1016/j.chembiol.2017.04.003. Cell Chem Biol. 2017. PMID: 28431224
Similar articles
-
Serine ADP-ribosylation reversal by the hydrolase ARH3.Elife. 2017 Jun 26;6:e28533. doi: 10.7554/eLife.28533. Elife. 2017. PMID: 28650317 Free PMC article.
-
HPF1/C4orf27 Is a PARP-1-Interacting Protein that Regulates PARP-1 ADP-Ribosylation Activity.Mol Cell. 2016 May 5;62(3):432-442. doi: 10.1016/j.molcel.2016.03.008. Epub 2016 Apr 7. Mol Cell. 2016. PMID: 27067600 Free PMC article.
-
Dual function of HPF1 in the modulation of PARP1 and PARP2 activities.Commun Biol. 2021 Nov 3;4(1):1259. doi: 10.1038/s42003-021-02780-0. Commun Biol. 2021. PMID: 34732825 Free PMC article.
-
The fast-growing business of Serine ADP-ribosylation.DNA Repair (Amst). 2022 Oct;118:103382. doi: 10.1016/j.dnarep.2022.103382. Epub 2022 Jul 29. DNA Repair (Amst). 2022. PMID: 35963141 Review.
-
ADP-ribose hydrolases: biological functions and potential therapeutic targets.Expert Rev Mol Med. 2024 Oct 8;26:e21. doi: 10.1017/erm.2024.17. Expert Rev Mol Med. 2024. PMID: 39375922 Free PMC article. Review.
Cited by
-
The Significance of Targeting Poly (ADP-Ribose) Polymerase-1 in Pancreatic Cancer for Providing a New Therapeutic Paradigm.Int J Mol Sci. 2021 Mar 29;22(7):3509. doi: 10.3390/ijms22073509. Int J Mol Sci. 2021. PMID: 33805293 Free PMC article. Review.
-
Rapid Detection and Signaling of DNA Damage by PARP-1.Trends Biochem Sci. 2021 Sep;46(9):744-757. doi: 10.1016/j.tibs.2021.01.014. Epub 2021 Mar 3. Trends Biochem Sci. 2021. PMID: 33674152 Free PMC article. Review.
-
AI26 inhibits the ADP-ribosylhydrolase ARH3 and suppresses DNA damage repair.J Biol Chem. 2020 Oct 2;295(40):13838-13849. doi: 10.1074/jbc.RA120.012801. Epub 2020 Aug 4. J Biol Chem. 2020. PMID: 32753484 Free PMC article.
-
PARP1 Hinders Histone H2B Occupancy at the NFATc1 Promoter to Restrain Osteoclast Differentiation.J Bone Miner Res. 2020 Apr;35(4):776-788. doi: 10.1002/jbmr.3927. Epub 2020 Jan 7. J Bone Miner Res. 2020. PMID: 31793068 Free PMC article.
-
Emerging roles of ADP-ribosyl-acceptor hydrolases (ARHs) in tumorigenesis and cell death pathways.Biochem Pharmacol. 2019 Sep;167:44-49. doi: 10.1016/j.bcp.2018.09.028. Epub 2018 Sep 27. Biochem Pharmacol. 2019. PMID: 30267646 Free PMC article. Review.
References
-
- Adamietz P. Poly(ADP-ribose) synthase is the major endogenous nonhistone acceptor for poly(ADP-ribose) in alkylated rat hepatoma cells. Eur. J. Biochem. 1987;169:365–372. - PubMed
-
- Bock F.J., Chang P. New directions in poly(ADP-ribose) polymerase biology. FEBS J. 2016;283:4017–4031. - PubMed
-
- Caldecott K.W. Protein ADP-ribosylation and the cellular response to DNA strand breaks. DNA Repair (Amst.) 2014;19:108–113. - PubMed
-
- Chapman J.D., Gagné J.P., Poirier G.G., Goodlett D.R. Mapping PARP-1 auto-ADP-ribosylation sites by liquid chromatography-tandem mass spectrometry. J. Proteome Res. 2013;12:1868–1880. - PubMed
-
- Cox J., Mann M. MaxQuant enables high peptide identification rates, individualized p.p.b.-range mass accuracies and proteome-wide protein quantification. Nat. Biotechnol. 2008;26:1367–1372. - PubMed
MeSH terms
Substances
Grants and funding
LinkOut - more resources
Full Text Sources
Other Literature Sources
Molecular Biology Databases
Miscellaneous