Understanding the FRET Signatures of Interacting Membrane Proteins
- PMID: 28188294
- PMCID: PMC5392676
- DOI: 10.1074/jbc.M116.764282
Understanding the FRET Signatures of Interacting Membrane Proteins
Abstract
FRET is an indispensable experimental tool for studying membrane proteins. Currently, two models are available for researchers to determine the oligomerization state of membrane proteins in a static quenching FRET experiment: the model of Veatch and Stryer, derived in 1977, and the kinetic theory-based model for intraoligomeric FRET, derived in 2007. Because of confinement in two dimensions, a substantial amount of FRET is generated by energy transfer between fluorophores located in separate oligomers in the two-dimensional bilayer. This interoligomeric FRET (also known as stochastic, bystander, or proximity FRET) is not accounted for in either model. Here, we use the kinetic theory formalism to describe the dependence of the FRET efficiency measured in an experiment (i.e. the "total apparent FRET efficiency") on the interoligomeric FRET due to random proximity within the bilayer and the intraoligomeric FRET resulting from protein-protein interactions. We find that data analysis with both models without consideration of the proximity FRET leads to incorrect conclusions about the oligomeric state of the protein. We show that knowledge of the total surface densities of fluorophore-labeled membrane proteins is essential for correctly interpreting the measured total apparent FRET efficiency. We also find that bulk, two-color, static quenching FRET experiments are best suited for the study of monomeric, dimerizing, or dimeric proteins but have limitations in discerning the order of larger oligomers. The theory and methodology described in this work will allow researchers to extract meaningful parameters from static quenching FRET measurements in biological membranes.
Keywords: cell surface receptor; fluorescence resonance energy transfer (FRET); mathematical modeling; membrane biophysics; membrane protein.
© 2017 by The American Society for Biochemistry and Molecular Biology, Inc.
Conflict of interest statement
The authors declare that they have no conflicts of interest with the contents of this article
Figures
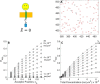
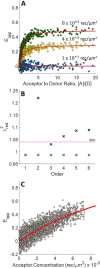
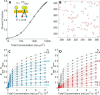
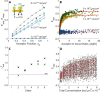
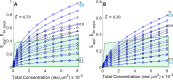
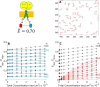
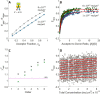
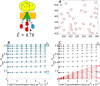
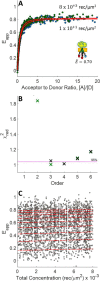
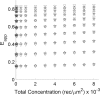
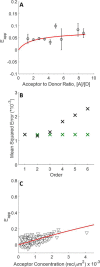
Similar articles
-
Membrane Protein Dimerization in Cell-Derived Lipid Membranes Measured by FRET with MC Simulations.Biophys J. 2020 Apr 21;118(8):1861-1875. doi: 10.1016/j.bpj.2020.03.011. Epub 2020 Mar 29. Biophys J. 2020. PMID: 32246901 Free PMC article.
-
Quantitative time domain analysis of lifetime-based Förster resonant energy transfer measurements with fluorescent proteins: Static random isotropic fluorophore orientation distributions.J Biophotonics. 2018 Jul;11(7):e201700366. doi: 10.1002/jbio.201700366. Epub 2018 May 2. J Biophotonics. 2018. PMID: 29582566
-
Enumeration of oligomerization states of membrane proteins in living cells by homo-FRET spectroscopy and microscopy: theory and application.Biophys J. 2007 May 1;92(9):3098-104. doi: 10.1529/biophysj.106.099424. Biophys J. 2007. PMID: 17416632 Free PMC article.
-
Fluorophores, environments, and quantification techniques in the analysis of transmembrane helix interaction using FRET.Biopolymers. 2015 Jul;104(4):247-64. doi: 10.1002/bip.22667. Biopolymers. 2015. PMID: 25968159 Free PMC article. Review.
-
Microscopic analysis of fluorescence resonance energy transfer (FRET).Methods Mol Biol. 2004;261:351-70. doi: 10.1385/1-59259-762-9:351. Methods Mol Biol. 2004. PMID: 15064469 Review.
Cited by
-
Determination of the size of lipid rafts studied through single-molecule FRET simulations.Biophys J. 2021 Jun 1;120(11):2287-2295. doi: 10.1016/j.bpj.2021.04.003. Epub 2021 Apr 20. Biophys J. 2021. PMID: 33864789 Free PMC article.
-
Single Proteoliposome High-Content Analysis Reveals Differences in the Homo-Oligomerization of GPCRs.Biophys J. 2018 Jul 17;115(2):300-312. doi: 10.1016/j.bpj.2018.05.036. Biophys J. 2018. PMID: 30021106 Free PMC article.
-
Quantitative characterization of tetraspanin 8 homointeractions in the plasma membrane.Biochem J. 2021 Oct 15;478(19):3643-3654. doi: 10.1042/BCJ20210459. Biochem J. 2021. PMID: 34524408 Free PMC article.
-
GPCR homo-oligomerization.Curr Opin Cell Biol. 2019 Apr;57:40-47. doi: 10.1016/j.ceb.2018.10.007. Epub 2018 Nov 16. Curr Opin Cell Biol. 2019. PMID: 30453145 Free PMC article. Review.
-
Quantitative FRET Microscopy Reveals a Crucial Role of Cytoskeleton in Promoting PI(4,5)P2 Confinement.Int J Mol Sci. 2021 Oct 29;22(21):11727. doi: 10.3390/ijms222111727. Int J Mol Sci. 2021. PMID: 34769158 Free PMC article.
References
-
- Chung I., Akita R., Vandlen R., Toomre D., Schlessinger J., and Mellman I. (2010) Spatial control of EGF receptor activation by reversible dimerization on living cells. Nature 464, 783–787 - PubMed
-
- Sako Y., Minoghchi S., and Yanagida T. (2000) Single-molecule imaging of EGFR signalling on the surface of living cells. Nat. Cell Biol. 2, 168–172 - PubMed
MeSH terms
Substances
Grants and funding
LinkOut - more resources
Full Text Sources
Other Literature Sources