Functional Coupling of Human Microphysiology Systems: Intestine, Liver, Kidney Proximal Tubule, Blood-Brain Barrier and Skeletal Muscle
- PMID: 28176881
- PMCID: PMC5296733
- DOI: 10.1038/srep42296
Functional Coupling of Human Microphysiology Systems: Intestine, Liver, Kidney Proximal Tubule, Blood-Brain Barrier and Skeletal Muscle
Erratum in
-
Corrigendum: Functional Coupling of Human Microphysiology Systems: Intestine, Liver, Kidney Proximal Tubule, Blood-Brain Barrier and Skeletal Muscle.Sci Rep. 2017 Mar 16;7:44517. doi: 10.1038/srep44517. Sci Rep. 2017. PMID: 28300206 Free PMC article. No abstract available.
Abstract
Organ interactions resulting from drug, metabolite or xenobiotic transport between organs are key components of human metabolism that impact therapeutic action and toxic side effects. Preclinical animal testing often fails to predict adverse outcomes arising from sequential, multi-organ metabolism of drugs and xenobiotics. Human microphysiological systems (MPS) can model these interactions and are predicted to dramatically improve the efficiency of the drug development process. In this study, five human MPS models were evaluated for functional coupling, defined as the determination of organ interactions via an in vivo-like sequential, organ-to-organ transfer of media. MPS models representing the major absorption, metabolism and clearance organs (the jejunum, liver and kidney) were evaluated, along with skeletal muscle and neurovascular models. Three compounds were evaluated for organ-specific processing: terfenadine for pharmacokinetics (PK) and toxicity; trimethylamine (TMA) as a potentially toxic microbiome metabolite; and vitamin D3. We show that the organ-specific processing of these compounds was consistent with clinical data, and discovered that trimethylamine-N-oxide (TMAO) crosses the blood-brain barrier. These studies demonstrate the potential of human MPS for multi-organ toxicity and absorption, distribution, metabolism and excretion (ADME), provide guidance for physically coupling MPS, and offer an approach to coupling MPS with distinct media and perfusion requirements.
Conflict of interest statement
The authors declare no competing financial interests.
Figures
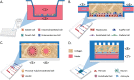
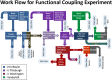
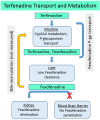
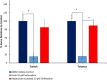
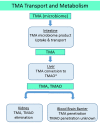
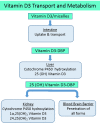
Similar articles
-
An Intestine/Liver Microphysiological System for Drug Pharmacokinetic and Toxicological Assessment.J Vis Exp. 2020 Dec 3;(166). doi: 10.3791/60184. J Vis Exp. 2020. PMID: 33346185
-
Introduction to a manuscript series on the characterization and use of microphysiological systems (MPS) in pharmaceutical safety and ADME applications.Lab Chip. 2020 Mar 17;20(6):1049-1057. doi: 10.1039/c9lc01168d. Lab Chip. 2020. PMID: 32073020
-
Emerging Kidney Models to Investigate Metabolism, Transport, and Toxicity of Drugs and Xenobiotics.Drug Metab Dispos. 2018 Nov;46(11):1692-1702. doi: 10.1124/dmd.118.082958. Epub 2018 Aug 3. Drug Metab Dispos. 2018. PMID: 30076203 Free PMC article. Review.
-
Multi-functional scaling methodology for translational pharmacokinetic and pharmacodynamic applications using integrated microphysiological systems (MPS).Integr Biol (Camb). 2017 Apr 18;9(4):290-302. doi: 10.1039/c6ib00243a. Integr Biol (Camb). 2017. PMID: 28267162 Free PMC article.
-
Microphysiological systems for ADME-related applications: current status and recommendations for system development and characterization.Lab Chip. 2020 Feb 7;20(3):446-467. doi: 10.1039/c9lc00857h. Epub 2020 Jan 14. Lab Chip. 2020. PMID: 31932816 Review.
Cited by
-
Links between Nutrition, Infectious Diseases, and Microbiota: Emerging Technologies and Opportunities for Human-Focused Research.Nutrients. 2020 Jun 19;12(6):1827. doi: 10.3390/nu12061827. Nutrients. 2020. PMID: 32575399 Free PMC article. Review.
-
Trimethylamine N-oxide promotes demyelination in spontaneous hypertension rats through enhancing pyroptosis of oligodendrocytes.Front Aging Neurosci. 2022 Aug 22;14:963876. doi: 10.3389/fnagi.2022.963876. eCollection 2022. Front Aging Neurosci. 2022. PMID: 36072486 Free PMC article.
-
Recent progress in translational engineered in vitro models of the central nervous system.Brain. 2020 Dec 5;143(11):3181-3213. doi: 10.1093/brain/awaa268. Brain. 2020. PMID: 33020798 Free PMC article. Review.
-
Human Epithelial Stem Cell-Derived Colonoid Monolayers as a Model to Study Shiga Toxin-Producing Escherichia coli-Host Interactions.Methods Mol Biol. 2021;2291:285-296. doi: 10.1007/978-1-0716-1339-9_13. Methods Mol Biol. 2021. PMID: 33704759
-
Construction and Functional Evaluation of a Three-Dimensional Blood-Brain Barrier Model Equipped With Human Induced Pluripotent Stem Cell-Derived Brain Microvascular Endothelial Cells.Pharm Res. 2022 Jul;39(7):1535-1547. doi: 10.1007/s11095-022-03249-3. Epub 2022 Apr 11. Pharm Res. 2022. PMID: 35411503 Free PMC article.
References
-
- Olson H. et al.. Concordance of the Toxicity of Pharmaceuticals in Humans and in Animals. Regulatory Toxicology and Pharmacology 32, 56–67 (2000). - PubMed
-
- Perel P. et al.. Comparison of treatment effects between animal experiments and clinical trials: systematic review. BMJ 334, 197, doi: 10.1136/bmj.39048.407928.BE (2007). - DOI - PMC - PubMed
Publication types
MeSH terms
Substances
Grants and funding
- R24 DK099803/DK/NIDDK NIH HHS/United States
- UH3 TR000504/TR/NCATS NIH HHS/United States
- K01 DK106323/DK/NIDDK NIH HHS/United States
- P30 CA047904/CA/NCI NIH HHS/United States
- P30 DK089502/DK/NIDDK NIH HHS/United States
- R01 AI080656/AI/NIAID NIH HHS/United States
- U19 AI116497/AI/NIAID NIH HHS/United States
- R01 DK026523/DK/NIDDK NIH HHS/United States
- P30 ES007033/ES/NIEHS NIH HHS/United States
- P30 DK056338/DK/NIDDK NIH HHS/United States
- U18 TR000552/TR/NCATS NIH HHS/United States
- KL2 TR002317/TR/NCATS NIH HHS/United States
- UH3 TR000491/TR/NCATS NIH HHS/United States
- KL2 TR000421/TR/NCATS NIH HHS/United States
- UH3 TR000505/TR/NCATS NIH HHS/United States
- UH3 TR000503/TR/NCATS NIH HHS/United States
- P30 AI036211/AI/NIAID NIH HHS/United States
- T32 DK007632/DK/NIDDK NIH HHS/United States
LinkOut - more resources
Full Text Sources
Other Literature Sources