Integrin-mediated mechanotransduction
- PMID: 27872252
- PMCID: PMC5119943
- DOI: 10.1083/jcb.201609037
Integrin-mediated mechanotransduction
Abstract
Cells can detect and react to the biophysical properties of the extracellular environment through integrin-based adhesion sites and adapt to the extracellular milieu in a process called mechanotransduction. At these adhesion sites, integrins connect the extracellular matrix (ECM) with the F-actin cytoskeleton and transduce mechanical forces generated by the actin retrograde flow and myosin II to the ECM through mechanosensitive focal adhesion proteins that are collectively termed the "molecular clutch." The transmission of forces across integrin-based adhesions establishes a mechanical reciprocity between the viscoelasticity of the ECM and the cellular tension. During mechanotransduction, force allosterically alters the functions of mechanosensitive proteins within adhesions to elicit biochemical signals that regulate both rapid responses in cellular mechanics and long-term changes in gene expression. Integrin-mediated mechanotransduction plays important roles in development and tissue homeostasis, and its dysregulation is often associated with diseases.
© 2016 Sun et al.
Figures
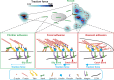
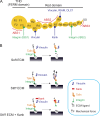
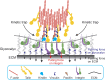
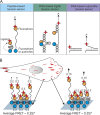
Similar articles
-
Talin - the master of integrin adhesions.J Cell Sci. 2017 Aug 1;130(15):2435-2446. doi: 10.1242/jcs.190991. Epub 2017 Jul 12. J Cell Sci. 2017. PMID: 28701514 Review.
-
Integrating actin dynamics, mechanotransduction and integrin activation: the multiple functions of actin binding proteins in focal adhesions.Eur J Cell Biol. 2013 Oct-Nov;92(10-11):339-48. doi: 10.1016/j.ejcb.2013.10.009. Epub 2013 Nov 4. Eur J Cell Biol. 2013. PMID: 24252517 Review.
-
Mechanical regulation of a molecular clutch defines force transmission and transduction in response to matrix rigidity.Nat Cell Biol. 2016 May;18(5):540-8. doi: 10.1038/ncb3336. Epub 2016 Apr 11. Nat Cell Biol. 2016. PMID: 27065098
-
Force-Directed "Mechanointeractome" of Talin-Integrin.Biochemistry. 2019 Nov 26;58(47):4677-4695. doi: 10.1021/acs.biochem.9b00442. Epub 2019 Aug 21. Biochemistry. 2019. PMID: 31393109 Review.
-
Molecular mechanisms of mechanotransduction in integrin-mediated cell-matrix adhesion.Exp Cell Res. 2016 Nov 15;349(1):85-94. doi: 10.1016/j.yexcr.2016.10.001. Epub 2016 Oct 6. Exp Cell Res. 2016. PMID: 27720950 Free PMC article. Review.
Cited by
-
The Mechanical Basis of Memory - the MeshCODE Theory.Front Mol Neurosci. 2021 Feb 25;14:592951. doi: 10.3389/fnmol.2021.592951. eCollection 2021. Front Mol Neurosci. 2021. PMID: 33716664 Free PMC article.
-
High Expression of THY1 in Intestinal Gastric Cancer as a Key Factor in Tumor Biology: A Poor Prognosis-Independent Marker Related to the Epithelial-Mesenchymal Transition Profile.Genes (Basel). 2023 Dec 24;15(1):28. doi: 10.3390/genes15010028. Genes (Basel). 2023. PMID: 38254918 Free PMC article.
-
Mechanical tumor microenvironment and transduction: cytoskeleton mediates cancer cell invasion and metastasis.Int J Biol Sci. 2020 Apr 27;16(12):2014-2028. doi: 10.7150/ijbs.44943. eCollection 2020. Int J Biol Sci. 2020. PMID: 32549750 Free PMC article. Review.
-
Talin dissociates from RIAM and associates to vinculin sequentially in response to the actomyosin force.Nat Commun. 2020 Jun 19;11(1):3116. doi: 10.1038/s41467-020-16922-1. Nat Commun. 2020. PMID: 32561773 Free PMC article.
-
Adaptable boronate ester hydrogels with tunable viscoelastic spectra to probe timescale dependent mechanotransduction.Biomaterials. 2019 Dec;223:119430. doi: 10.1016/j.biomaterials.2019.119430. Epub 2019 Aug 13. Biomaterials. 2019. PMID: 31493696 Free PMC article.
References
-
- Alexandrova A.Y., Arnold K., Schaub S., Vasiliev J.M., Meister J.J., Bershadsky A.D., and Verkhovsky A.B.. 2008. Comparative dynamics of retrograde actin flow and focal adhesions: formation of nascent adhesions triggers transition from fast to slow flow. PLoS One. 3:e3234 10.1371/journal.pone.0003234 - DOI - PMC - PubMed
Publication types
MeSH terms
Substances
Grants and funding
LinkOut - more resources
Full Text Sources
Other Literature Sources