IRGB10 Liberates Bacterial Ligands for Sensing by the AIM2 and Caspase-11-NLRP3 Inflammasomes
- PMID: 27693356
- PMCID: PMC5074697
- DOI: 10.1016/j.cell.2016.09.012
IRGB10 Liberates Bacterial Ligands for Sensing by the AIM2 and Caspase-11-NLRP3 Inflammasomes
Abstract
The inflammasome is an intracellular signaling complex, which on recognition of pathogens and physiological aberration, drives activation of caspase-1, pyroptosis, and the release of the pro-inflammatory cytokines IL-1β and IL-18. Bacterial ligands must secure entry into the cytoplasm to activate inflammasomes; however, the mechanisms by which concealed ligands are liberated in the cytoplasm have remained unclear. Here, we showed that the interferon-inducible protein IRGB10 is essential for activation of the DNA-sensing AIM2 inflammasome by Francisella novicida and contributed to the activation of the LPS-sensing caspase-11 and NLRP3 inflammasome by Gram-negative bacteria. IRGB10 directly targeted cytoplasmic bacteria through a mechanism requiring guanylate-binding proteins. Localization of IRGB10 to the bacterial cell membrane compromised bacterial structural integrity and mediated cytosolic release of ligands for recognition by inflammasome sensors. Overall, our results reveal IRGB10 as part of a conserved signaling hub at the interface between cell-autonomous immunity and innate immune sensing pathways.
Keywords: GBP2; GBP5; GBPs; LPS; caspase-1; cell-autonomous immunity; immunity-related GTPases; innate immunity; interferons; non-canonical inflammasome.
Copyright © 2016 Elsevier Inc. All rights reserved.
Figures
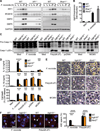
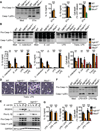
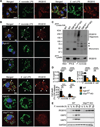
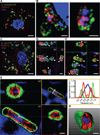
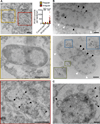
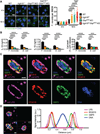
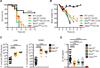
Comment in
-
IRGB10 Exposes Bacteria's Intimate Secrets.Dev Cell. 2016 Oct 10;39(1):7-8. doi: 10.1016/j.devcel.2016.09.026. Dev Cell. 2016. PMID: 27728782
Similar articles
-
IFN-γ extends the immune functions of Guanylate Binding Proteins to inflammasome-independent antibacterial activities during Francisella novicida infection.PLoS Pathog. 2017 Oct 2;13(10):e1006630. doi: 10.1371/journal.ppat.1006630. eCollection 2017 Oct. PLoS Pathog. 2017. PMID: 28968459 Free PMC article.
-
The role of NLRP3 and AIM2 in inflammasome activation during Brucella abortus infection.Semin Immunopathol. 2017 Feb;39(2):215-223. doi: 10.1007/s00281-016-0581-1. Epub 2016 Jul 12. Semin Immunopathol. 2017. PMID: 27405866 Free PMC article. Review.
-
Francisella Inflammasomes: Integrated Responses to a Cytosolic Stealth Bacterium.Curr Top Microbiol Immunol. 2016;397:229-56. doi: 10.1007/978-3-319-41171-2_12. Curr Top Microbiol Immunol. 2016. PMID: 27460813 Review.
-
DNA-sensing inflammasomes: regulation of bacterial host defense and the gut microbiota.Pathog Dis. 2016 Jun;74(4):ftw028. doi: 10.1093/femspd/ftw028. Epub 2016 Apr 6. Pathog Dis. 2016. PMID: 27056948 Free PMC article. Review.
-
Interferon-inducible guanylate-binding proteins at the interface of cell-autonomous immunity and inflammasome activation.J Leukoc Biol. 2017 Jan;101(1):143-150. doi: 10.1189/jlb.4MR0516-223R. Epub 2016 Jul 14. J Leukoc Biol. 2017. PMID: 27418355 Free PMC article. Review.
Cited by
-
Interferons: Tug of War Between Bacteria and Their Host.Front Cell Infect Microbiol. 2021 Mar 10;11:624094. doi: 10.3389/fcimb.2021.624094. eCollection 2021. Front Cell Infect Microbiol. 2021. PMID: 33777837 Free PMC article. Review.
-
Mitochondrial VDAC1: A Potential Therapeutic Target of Inflammation-Related Diseases and Clinical Opportunities.Cells. 2022 Oct 10;11(19):3174. doi: 10.3390/cells11193174. Cells. 2022. PMID: 36231136 Free PMC article. Review.
-
The regulation of the ZBP1-NLRP3 inflammasome and its implications in pyroptosis, apoptosis, and necroptosis (PANoptosis).Immunol Rev. 2020 Sep;297(1):26-38. doi: 10.1111/imr.12909. Epub 2020 Jul 29. Immunol Rev. 2020. PMID: 32729116 Free PMC article. Review.
-
A Rapid Caspase-11 Response Induced by IFNγ Priming Is Independent of Guanylate Binding Proteins.iScience. 2020 Sep 29;23(10):101612. doi: 10.1016/j.isci.2020.101612. eCollection 2020 Oct 23. iScience. 2020. PMID: 33089101 Free PMC article.
-
NINJ1 mediates inflammatory cell death, PANoptosis, and lethality during infection conditions and heat stress.Nat Commun. 2024 Feb 26;15(1):1739. doi: 10.1038/s41467-024-45466-x. Nat Commun. 2024. PMID: 38409108 Free PMC article.
References
-
- Bernstein-Hanley I, Balsara ZR, Ulmer W, Coers J, Starnbach MN, Dietrich WF. Genetic analysis of susceptibility to Chlamydia trachomatis in mouse. Genes and immunity. 2006a;7:122–129. - PubMed
-
- Bernstein-Hanley I, Coers J, Balsara ZR, Taylor GA, Starnbach MN, Dietrich WF. The p47 GTPases Igtp and Irgb10 map to the Chlamydia trachomatis susceptibility locus Ctrq-3 and mediate cellular resistance in mice. Proceedings of the National Academy of Sciences of the United States of America. 2006b;103:14092–14097. - PMC - PubMed
Publication types
MeSH terms
Substances
Grants and funding
LinkOut - more resources
Full Text Sources
Other Literature Sources
Molecular Biology Databases
Research Materials
Miscellaneous