A Portrait of the Human Organelle Proteome In Space and Time during Cytomegalovirus Infection
- PMID: 27641956
- PMCID: PMC5083158
- DOI: 10.1016/j.cels.2016.08.012
A Portrait of the Human Organelle Proteome In Space and Time during Cytomegalovirus Infection
Abstract
The organelles within a eukaryotic host are manipulated by viruses to support successful virus replication and spread of infection, yet the global impact of viral infection on host organelles is poorly understood. Integrating microscopy, subcellular fractionation, mass spectrometry, and functional analyses, we conducted a cell-wide study of organelles in primary fibroblasts throughout the time course of human cytomegalovirus (HCMV) infection. We used label-free and isobaric-labeling proteomics to characterize nearly 4,000 host and 100 viral proteins, then classified their specific subcellular locations over time using machine learning. We observed a global reorganization of proteins across the secretory pathway, plasma membrane, and mitochondria, including reorganization and processing of lysosomal proteins into distinct subpopulations and translocations of individual proteins between organelles at specific time points. We also demonstrate that MYO18A, an unconventional myosin that translocates from the plasma membrane to the viral assembly complex, is necessary for efficient HCMV replication. This study provides a comprehensive resource for understanding host and virus biology during HCMV pathogenesis.
Keywords: HCMV; cytomegalovirus; organelle; proteomics; spatial.
Copyright © 2016 Elsevier Inc. All rights reserved.
Figures
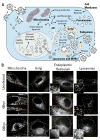
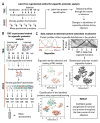
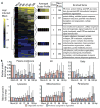
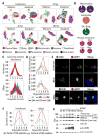
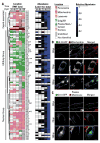
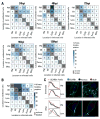
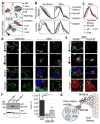
Similar articles
-
HCMV strain- and cell type-specific alterations in membrane contact sites point to the convergent regulation of organelle remodeling.J Virol. 2024 Nov 19;98(11):e0109924. doi: 10.1128/jvi.01099-24. Epub 2024 Oct 31. J Virol. 2024. PMID: 39480111
-
The life cycle and pathogenesis of human cytomegalovirus infection: lessons from proteomics.Expert Rev Proteomics. 2014 Dec;11(6):697-711. doi: 10.1586/14789450.2014.971116. Epub 2014 Oct 18. Expert Rev Proteomics. 2014. PMID: 25327590 Free PMC article.
-
TRANSPIRE: A Computational Pipeline to Elucidate Intracellular Protein Movements from Spatial Proteomics Data Sets.J Am Soc Mass Spectrom. 2020 Jul 1;31(7):1422-1439. doi: 10.1021/jasms.0c00033. Epub 2020 May 29. J Am Soc Mass Spectrom. 2020. PMID: 32401031 Free PMC article.
-
Virus-host protein interactions as footprints of human cytomegalovirus replication.Curr Opin Virol. 2022 Feb;52:135-147. doi: 10.1016/j.coviro.2021.11.016. Epub 2021 Dec 16. Curr Opin Virol. 2022. PMID: 34923282 Free PMC article. Review.
-
Exploring and Exploiting Proteome Organization during Viral Infection.J Virol. 2017 Aug 24;91(18):e00268-17. doi: 10.1128/JVI.00268-17. Print 2017 Sep 15. J Virol. 2017. PMID: 28679763 Free PMC article. Review.
Cited by
-
Semi-Supervised Non-Parametric Bayesian Modelling of Spatial Proteomics.Ann Appl Stat. 2022 Dec 1;16(4):22-aoas1603. doi: 10.1214/22-AOAS1603. eCollection 2022 Dec 1. Ann Appl Stat. 2022. PMID: 36507469 Free PMC article.
-
The metabolites NADP+ and NADPH are the targets of the circadian protein Nocturnin (Curled).Nat Commun. 2019 May 30;10(1):2367. doi: 10.1038/s41467-019-10125-z. Nat Commun. 2019. PMID: 31147539 Free PMC article.
-
Recent Advancements in Subcellular Proteomics: Growing Impact of Organellar Protein Niches on the Understanding of Cell Biology.J Proteome Res. 2024 Aug 2;23(8):2700-2722. doi: 10.1021/acs.jproteome.3c00839. Epub 2024 Mar 7. J Proteome Res. 2024. PMID: 38451675 Free PMC article. Review.
-
Systems Virology and Human Cytomegalovirus: Using High Throughput Approaches to Identify Novel Host-Virus Interactions During Lytic Infection.Front Cell Infect Microbiol. 2020 Jun 10;10:280. doi: 10.3389/fcimb.2020.00280. eCollection 2020. Front Cell Infect Microbiol. 2020. PMID: 32587832 Free PMC article. Review.
-
A slowly cleaved viral signal peptide acts as a protein-integral immune evasion domain.Nat Commun. 2021 Apr 6;12(1):2061. doi: 10.1038/s41467-021-21983-x. Nat Commun. 2021. PMID: 33824318 Free PMC article.
References
Publication types
MeSH terms
Substances
Grants and funding
LinkOut - more resources
Full Text Sources
Other Literature Sources
Miscellaneous